Peptide mass fingerprinting (PMF) is a mass spectrometry protein identification technique based on peptide mass detection and is useful for differential expression analysis in the search for drug targets and for biomarker discovery.1,2 The efficiency of PMF experiments can be related to the number of proteolytic peptide fragments that are identified. Maintaining the protein identification confidence level becomes more challenging when concentrations of protein in a sample are reduced, as in the case of a limited number of cells or tissue. The problem of reduced peptide detection is further exacerbated by proton competition between the high- and low-abundance analyte ions.3,4 This can result in ion suppression and loss of detection of low-abundance peptides or proteins, thus reducing the sequence coverage for the protein of interest. Small-scale liquid chromatography separation can significantly enhance the detection of peptide and protein samples during MS analysis. 5–7 The LC separation partitions the coeluting low- and high-abundance analyte ions and reduces ion suppression effects.8 Ultimately, this increases the sequence coverage for the protein of interest. A variety of small-scale LC separation modes can be performed either separately or in combination and include affinity capture, ion exchange, size exclusion chromatofocusing, and reversed phase.9–11
The PiezoLC (MicroFab Technologies, Inc., Plano, TX, patent pending) is an inkjet system that delivers microvolumes (0.1–100 nL) of chromatographically separated proteolysed peptides onto matrix-assisted laser desorption ionizationtime of flight (MALDI-TOF) MS targets for subsequent MS analysis. A porous polymer monolith is located within the glass capillary of the inkjet dispensing device to provide chromatographic separation of peptides. The peptide sample is loaded onto the integrated chromatography column (reversed phase) and an elution buffer separates the peptides as the buffer passes through the column. The eluted peptides exit the orifice of the piezoelectric device in the form of drops and land on a MALDI-TOF MS target plate, preserving the chromatographic separation. The analysis of the peptides can then be performed at a later date and multiple times.
The quantity of protein and peptide materials available for PMF analysis is often limited, and miniaturization of the fluid handling for the mapping process is desired. The PiezoLC inkjet device utilizes a polymeric monolithic column and requires a small volume (<10 μL) of proteolysed material. Methacrylate-based polymeric separation media do not require the packing of beads or frits, which are difficult to incorporate in microfluidic devices and can interfere with fluid flow.12 The large pore size of the polymeric monolithic material results in low backpressure, which is important for the efficient operation of the system’s dispensing device. Polymerization by UV irradiation enables patterning and positioning of the monolithic column. Multiple chemistries/functions can be combined in one monolith, i.e., strong cation exchange (SCX) and reversed phase (RP). Additionally, these columns are robust and have fast separation due to rapid convective mass transfer.13
The PiezoLC can be a useful tool for the identification and characterization of proteins from finite samples. The chromatographic separation of peptides by the inkjet system can reduce ion suppression and improve the resolution of MALDI-TOF MS analysis for applications such as peptide mass fingerprinting. The separation of tryptic digests of bovine serum albumin (BSA) resulted in a larger number of peptides identified in comparison to the control. This resulted in a higher degree of amino acid sequence coverage and improved protein identification.
Methods
BSA in solution digest
In-solution digests of BSA were performed as described by Kinter et al.14 The BSA was mixed with 20 μg of trypsin (Promega, Madison, WI) and was incubated at 37 °C overnight.
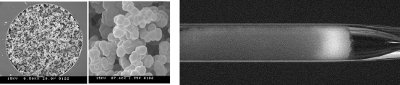
Figure 1 - Left: Cross-section and porous structure of monolith. Right: fluorescein isothiocyanate (FITC)-labeled angiotensin II captured on monolith in glass capillary.
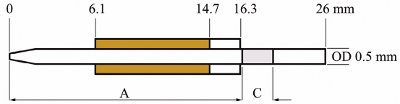
Figure 2 - Schematic of the PiezoLC microdispensing device showing location of monolithic column “C” in proximity to orifice at distance “A.”
Monolithic columns
- Borosilicate glass capillary vinylization. Monolithic poly(butylmethacrylate-co-ethylene dimethacrylate) capillary columns were prepared as described by Lee et al.15 The internal wall surface of the PiezoLC borosilicate glass capillaries was vinylized to enable the covalent attachment of the monolith.
- Polymerization mixture. The vinylized borosilicate glass capillaries were masked using opaque electrical tape and filled with the following polymerization mixture: 16% (v/v) ethylene dimethacrylate, 24% (v/v) butylmethacrylate, 59% (v/v) 1-decanol, and 1.0% (v/v) 2,2-dimethoxy-2-phenylacetophenone (Sigma-Aldrich, St. Louis, MO). The filled capillaries were irradiated for 15 min using a 365-nm 15-mW/cm2 UV light source ELC 4001 (Electro-Lite Corp., Danbury, CT). The pore size of the RP mode polymeric monolith was measured to be 2.2 μm.
- PiezoLC microdispensing device assembly. The glass capillaries containing monoliths were used in the assembly of the PiezoLC inkjet microdispensing devices (Figure 1). Various dispensing tests were performed with the monolithic column in different locations relative to the dispensing orifice. The location of the column in the glass capillary shown in Figure 2 was based on the least amount of interference with drop formation and dispensing.
Elution experiments
- Matrix solution. Recrystallized α-cyano-4-hydroxycinnamic acid (αCHCA) (LaserBio Labs, Sophia-Antipolis, France) was dissolved in a 35% (w/v) solution containing equal parts of 1-propanol, methanol, 1-butanol, and acetonitrile (Sigma-Aldrich). The αCHCA solution containing 0.1% (v/v) trifluoroacetic acid (TFA) was deposited onto a stainless steel MALDI target plate (PN DE1271TA, Shimadzu Scientific Instruments, Columbia, MD) at 100 nL per spot using a piezoelectric dispensing device having a 55-μm orifice diameter (PN MJ-AT-01-55, MicroFab Technologies, Inc.).
- Column loading. The PiezoLC device was flushed with 200 μL of 70% (v/v) acetonitrile and 0.1% (v/v) TFA, followed by a 200-μL flush of 0.1% (v/v) TFA. The BSA digest (50 fmol/μL) was loaded onto the column. The column was then flushed with 200 μL of 0.1% (v/v) TFA.
- Stepwise elution. A stepwise gradient elution in 5% steps between 5% and 70% was performed using a solution containing equal parts of 1-propanol, methanol, 1-butanol, and acetonitrile (Sigma-Aldrich) and 0.1% (v/v) TFA (EBD1 elution buffer). A volume of 100 nL of eluent was overprinted onto 12 matrix spots per elution step at a flow rate of 6.0 μL/min. The control was an isocratic elution of the BSA digest using a 70% concentration of EBD1 elution buffer.
- MALDI-TOF MS and PMF. MALDI-TOF MS analysis was performed in reflectron mode using an Axima CFR MALDI-TOF MS (Shimadzu Scientific Instruments) with 10 laser shots per profile and 100 profiles per sample spot. Spectra were internally calibrated using peptide masses of 1439.8117 Da and 2045.0279 Da. The peak smoothing method was Savitsky-Golay with peak picking method gradient–centroid. Peak lists of monoisotopic masses generated by the Axima software were uploaded to the MASCOT PMF search engine.16 The SwissProt database was searched using the variable modification carbamidomethyl (C) and peptide tolerance of ±0.2 Da.
Results
MALDI-TOF MS spectra
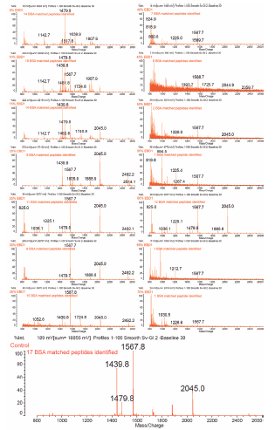
Figure 3 - MALDI-TOF MS spectra of the BSA digest eluted from the PiezoLC device during a stepwise gradient elution of 5%–70% concentration. The number of BSA matched peptides during PMF is indicated. The bottom spectrum is from the 70% isocratic elution control.
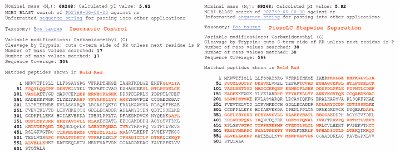
Figure 4 - MASCOT search results displaying matched peptides for Bos taurus from the PiezoLC isocratic elution and stepwise gradient separation of a BSA digest.
The LC separation of the BSA digest peptides is visible when comparing the MS spectra for each elution buffer concentration in Figure 3. This figure displays the MALDI-TOF MS spectra from each gradient elution of BSA digest from the PiezoLC device using EBD1 elution buffer 5%–70% concentration.
The sequence coverage and the number of peptides identified for the isocratic-eluted control was 30% and 17, respectively. The PiezoLC separation of the same BSA digest using a stepwise gradient elution resulted in sequence coverage of 56% with 38 peptides being identified (Figure 4). This is an 87% increase in the sequence coverage and 124% increase in the number of peptides identified over the control percentage and number. No significant matches to BSA peptides were identified during the MASCOT search of the mass peak lists from the stepwise gradient elutions of 50%, 55%, 65%, and 70%. These results were consistent with other PiezoLC BSA digest elution experiments.
Conclusion
A drop-on-demand piezoelectric device containing a polymeric monolithic column has been used to dispense eluents of proteolysed material onto a MALDI-TOF MS target for PMF. The LC separation of the BSA digest peptides generated during stepwise gradient elution improved sequence coverage and protein identification. This microfluidic approach to protein digest sample separation can significantly facilitate the PMF of low-concentration proteins and offers a new instrument for proteomic analysis.
References
- Zolg, J.W.; Langen, H. How industry is approaching the search for new diagnostic markers and biomarkers. Mol. Cell Proteom.2004, 3, 345–54.
- Chong, B.E.; Hamler, R.L.; Lubman, D.M.; Ethier, S.P.; Rosenspire, A.J.; Miller, F.R. Differential screening and mass mapping of proteins from premalignant and cancer cell lines using nonporous reversed-phase HPLC coupled with mass spectrometric analysis. Anal. Chem.2001, 73, 1219–27.
- Kratzer, R.; Eckerskorn, C.; Karas, M.; Lottspeich, M. Suppression effects in enzymatic peptide ladder sequencing using ultraviolet-matrix assisted laser desorption/ionization-mass spectrometry. Electrophoresis1998, 19, 1910–19.
- Knochenmuss, R.; Stortelder, A.; Breuker, K.; Zenobi, R. Secondary ion-molecule reactions in matrixassisted laser desorption/ionization. J. Mass Spectrom. 2000, 35, 1237–45.
- Shen, Y.; Zhao, R.; Berger, S.J.; Anderson, G.A.; Rodriguez, N.; Smith, R.D. High-efficiency nanoscale liquid chromatography coupled on-line with mass spectrometry using nanoelectrospray ionization for proteomics. Anal. Chem.2002, 74, 4235–49.
- Shen, Y.; Smith, R.D. Advanced nanoscale separations and mass spectrometry for sensitive high-throughput proteomics. Expert Rev. Proteom. 2005, 2, 431–47.
- Shen, Y.; Smith, R.D. Proteomics based on high- efficiency capillary separations. Electrophoresis2002, 23, 3106–24.
- Mallet, C.R.; Lu, Z.; Mazzeo, J.R. A study of ion suppression effects in electrospray ionization from mobile phase additives and solid-phase extracts. Rapid Commun. Mass Spectrom. 2004, 18, 49–58.
- Lee, W.C.; Lee, K.H. Applications of affinity chromatography in proteomics. Anal. Biochem.2004, 324, 1–10.
- Wang, H.; Hanash, S. Intact-protein based sample preparation strategies for proteome analysis in combination with mass spectrometry. Mass Spectrom. Rev.2005, 24, 413–26.
- Stroink, T.; Schravendijk, P.; Wiese, G.; Teeuwsen, J.; Lingeman, H.; Waterval, J.C.; Bult, A.; de Jong, G.J.; Underberg, W.J. On-line coupling of size-exclusion chromatography and capillary zone electrophoresis via a reversed-phase C18 trapping column for the determination of peptides in biological samples. Electrophoresis 2003, 24, 1126–34.
- Benes, M.J.; Horak, D.; Svec, F. Methacrylatebased chromatographic media. J. Sep. Sci.2005, 28, 1855–75.
- Jungbauer, A.; Hahn, R. Monoliths for fast bioseparation and bioconversion and their applications in biotechnology. J. Sep. Sci. 2004, 27, 767–78.
- Kinter, M.; Sherman, N.E. Protein Sequencing and Identification Using Tandem Mass Spectrometry. John Wiley & Sons, Inc.: New York, NY, 2000; pp 160–4.
- Lee, D.; Svec, F.; Frechet, J.M. Photopolymerized monolithic capillary columns for rapid micro highperformance liquid chromatographic separation of proteins. J. Chromatogr. A 2004, 1051, 53–60.
- http://www.matrixscience.com/cgi/search_form.pl? FORMVER=2&SEARCH=PMF.
Mr. Cooley is Biomedical Scientist, Dr. Chen is Senior Scientist, and Dr. Wallace is Vice President, MicroFab Technologies, Inc., 1104 Summit Ave., Ste. 110, Plano, TX 75074, U.S.A.; tel.: 972-578-8076, ext. 17; fax: 972-423-2438; e-mail: [email protected]. Mrs. Hopwood is Program Leader, Mass Spectrometry, and Dr. Gooley is Chief Scientific Officer, Proteome Systems, Ltd., North Ryde, NSW, Australia. Dr. Svec is Lead Scientist, The Molecular Foundry, Lawrence Berkeley National Laboratory, Berkeley, CA, U.S.A. Support was provided by NIH grant number 2R44 RR104939 and Materials Sciences and Engineering Division of the U.S. Department of Energy under contract number DE-AC02-05CH11231.