Investigations for the determination of drugs in real samples, while gaining in importance, are limited by time and sample throughput. In response to this, the authors developed an automated solid-phase extraction (SPE) procedure using a liquid-handling platform with a positive pressure unit. This automated SPE was validated after the manual SPE process. HPLC/MS was used to determine drug concentration. The results showed that 1) automation offers a precise and robust system for sample preparation and 2) automated SPE is suitable for routine analysis with less manual processing and fewer operator errors.
Introduction
Each year, 1.7 million people in the U.S. are diagnosed with cancer; of these, approximately 580,000 will die from the disease.1,2 Demand for pharmacological investigations, specifically the determination of drugs in real samples, has become increasingly important to optimize cancer treatment.3
Currently available automated SPE methods have limitations or do not provide high throughput. Allanson et al.4 and Marinova et al.5 presented a highly precise automated SPE process performed on a liquid handling platform that used a vacuum module for sample processing. However, automated SPE systems working with vacuum have a number of disadvantages, including a maximum pressure difference of 1 bar on the columns and imbalanced, or irregular, sample processing. In the latter case, various SPE columns are emptied at different speeds. This is problematic because depleted columns can cause a reduction in vacuum, leading to longer sample processing times due to a slower sample flow rate. It is important that the processing time for all columns is equal to ensure consistent sample handling and reliable results.6 For complete sample processing, vacuum is often applied to the columns for a longer period of time, even if they are already empty; however, this extended drying time may have a negative impact on the SPE.
The positive pressure unit used in this study overcomes these drawbacks because it is able to operate at a higher pressure, which ranges from 0.1 to 7 bar and guarantees constant pressure on all columns. This results in defined and steady pressure being applied over a specified time. Moreover, the positive pressure unit can be controlled by liquid handlers to achieve automated SPE. The system also provides conditioning and rinsing of the columns.6
Manual and automated SPE procedures
Manual and automated SPE were compared for the quantification of the cytotoxic drug cyclophosphamide in biological samples. Cyclophosphamide is used in chemotherapy and in the treatment of scleroderma lung disease and multiple sclerosis.7–9 Manual SPE with vacuum was used to extract cyclophosphamide. The automated system consisted of a Biomek NX liquid handler (Beckman Coulter, Brea, Calif.) with a Span-8 pipetting head and positive pressure unit for sample processing (amplius GmbH, Rostock, Germany). The system provides precise and robust SPE, is adaptable to different methods, requires less manual labor and reduces errors compared to manual processing errors.
Materials and methods
Manual solid-phase extraction
A Chromabond vacuum chamber (Macherey-Nagel, Düren, Germany) and Strata C18-E cartridges (Phenomenex, Torrance, Calif.) were used for manual liquid handling. Cyclophosphamide (Sigma-Aldrich, St. Louis, Mo.) was dissolved in Dulbecco’s Modified Eagle’s medium (DMEM) supplied with fetal calf serum (FCS), penicillin/streptomycin and glutamin (Sigma-Aldrich). To extract cyclophosphamide using the SPE process, Strata C18-E cartridges were conditioned with 2 mL of gradient-grade methanol ROTISOLV HPLC (Roth, Karlsruhe, Germany). The conditioning step was followed by preequilibration with 2 mL of Milli-Q organic-free water (Millipore, Bedford, Mass.). Subsequently, 1 mL of cyclophosphamide dissolved in DMEM was added and passed slowly through the column. Cartridges were washed with 2 mL of Milli-Q organic-free water and dried for 10 minutes. Finally, cyclophosphamide was eluted twice with 500 μL gradient-grade methanol ROTISOLV HPLC and samples were diluted accordingly.
Automated solid-phase extraction
Figure 1 – Positive pressure unit for automated solid-phase extraction shown with a 96-deep-well plate for cyclophosphamide extraction working with positive pressure.The system was validated with two different plates: 24 columns, which were stacked in an adapter plate, and a 96-deep-well collection plate (Phenomenex). These two plates were placed into the positive pressure unit (Figure 1). Positive pressure forced the liquids through the cartridges. The automated SPE steps were done in accordance with the manual procedure. An appropriate sample dilution was included in the automated process.
HPLC/MS
Table 1 – HPLC/MS method parameters for cyclophosphamide analysis
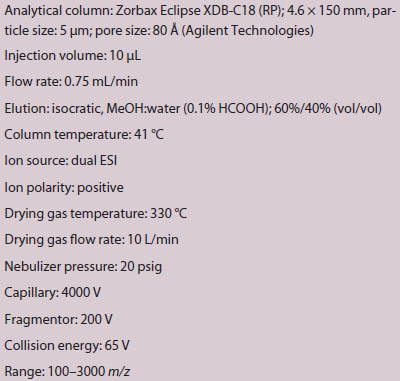
An Agilent 1200 series system (Agilent Technologies, Santa Clara, Calif.) equipped with a LC/MS TOF, high-performance autosampler, binary pump and thermostated column compartment was used for the chromatographic separation. Specific parameters of the HPLC/MS method for cyclophosphamide determination are shown in Table 1. Cyclophosphamide detection was done with an Agilent autosampler SL, LC/MS TOF and electrospray ionization (ESI) interface. The retention time of cyclophosphamide was 3.59 minutes and 2.36 minutes for caffeine as the internal standard. In the positive ion mode, the molar mass was 261.03 g/mol for cyclophosphamide and 195.09 g/mol for caffeine. Qualitative and quantitative analyses were performed with Agilent MassHunter workstation software.
Assay validation
For SPE method validation, the linear calibration curve ranged from 5 to 500 ppb (μg/L). To verify the method, DMEM containing 200 ppb of cyclophosphamide and 800 ppb of caffeine was used. One sample was quantified 10 times to assess measuring precision. To determine repeatability precision, 25 samples were analyzed in one day. The limit of detection (LOD) and limit of quantification (LOQ) were determined on the basis of 10 blanks measured on the same day. LOD was calculated based on the signal-to-noise ratio. LOQ is the lowest measurable concentration of the analyte.
To test the within-day precision, 10 samples for the manual procedure and 24 or 96 samples for the automated process were produced and analyzed each day for five days. For the between-day precision, samples were analyzed on five different days. The samples were split into five parts and refrigerated and measured over five consecutive days. Hence, 210 samples were quantified using both manual and automated SPE. Data are presented as mean value (MV%) and relative standard deviation (RSD%).
Results
Table 2 – Between-day precision for 10 samples
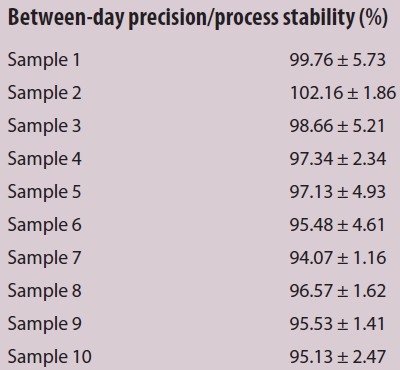
The calibration curve is linear in the range from 5 to 500 ppb (y = 0.0015 · x + 0.0022) with a correlation coefficient of R = 0.998 (Figure 2). To validate the methods, 200 ppb was used in both procedures and set as 100% for final comparison of the manual and automated processes. The manual process was first validated and set as the comparison reference. The between-day precision was averaged at 97.18 ± 3.13 % (Table 2). The LOD was 6.85 μg/L and the LOQ was 13.12 μg/L for the manual process. For the automated process, the LOD was 6.26 μg/L and the LOQ was 6.77 μg/L (Table 3). The measuring precision for the manual process was 91.58 ± 2.61% and 96.09 ± 1.44% for the automated procedure. In addition, the manual sample preparation demonstrated a repeatability precision of 95.26 ± 4.14%; the repeatability precision of the automated process was 97.63 ± 4.39% with the 24-column adapter plate and 74.35 ± 5.89% with the 96-deep-well plate, respectively. The automated process with the 24-column adapter plate had a higher repeatability precision than the manual process, but the opposite was true with the 96-deep-well plate. Possible reasons are that foam developed in the waste reservoir during sample processing, i.e., foam rose up from the waste reservoir to the columns, negatively affecting the adsorbate and the results. Consequently, sample incubation time in the columns was reduced from 7 seconds (with the 24-column adapter plate) to 3 seconds (with the 96-deep-well plate) to minimize foam development.
Figure 2 – Calibration curve. Calibration function: y = 0.0015 · x + 0.0022; R2 = 0.998; analysis via HPLC/MS.Table 3 – Comparison of manual and automated SPE
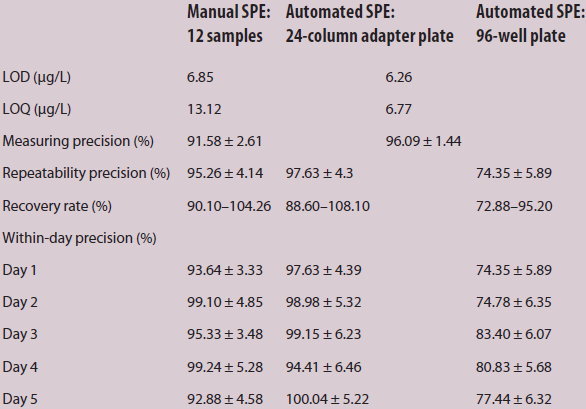
The recovery rate for the automated process was in a slightly lower range (24-column adapter plate: 88.60–108.10%, MV: 98.35%; 96-well plate: 72.88–95.20%, MV: 84.04%) compared to the manual procedure (90.10–104.26%, MV: 97.18%). The within-day precision for the manual SPE was 96.04 ± 4.30% for all five days. In contrast, the automated process with the 24-column adapter plates achieved a higher within-day precision (98.04 ± 5.52%) and the 96-deep-well plate a lower within-day precision (78.16 ± 6.06 μg/L). The presence of foam may have been a factor in the lower within-day precision with the 96-deep-well plates. Further, the shorter incubation time of the sample within the column influenced the adsorption process. In the automated procedure, a maximum of two plates (24 or 96 samples) can be processed in one day because of the time-consuming sample preparation, decontamination, liquid handling and detection method. Therefore, optimization of the automated SPE can be achieved by 1) using a larger waste reservoir and removing the foam and 2) curtailing the liquid handling process and the quantification method.
Discussion
The findings presented here demonstrate the success of the automated SPE with the positive pressure unit. The results show that the automated process with the 24-column adapter and 96-well plate are in an acceptable range and can replace the manual sample preparation process. Automated and semiautomated SPE methods using vacuum for sample processing6,10 are suitable for routine use. In Ref. 11, Chen et al. presented a fully automated solid-phase extraction method for drug screening purposes and compared automated to manually generated results.
In the current study, automated SPE was based on positive pressure and manual SPE was based on vacuum. Consistent with this, Chen et al. obtained better reproducibility, with the automated process.11
Conclusion
The positive pressure unit described in this article can replace the manual process as well as vacuum systems while achieving reliable results. Advantages include higher applicable pressure on the columns than vacuum systems, uniform pressure over all the columns and complete and consistent sample processing. Consequently, the automated SPE method with positive pressure has a defined working time due to complete sample processing. In contrast with the vacuum method, the processing time needs to be extended to ensure fully processed samples. During this extended processing time, already emptied columns dry out, which negatively impacts the SPE and the results generated.
In conclusion, the positive pressure unit delivers optimized automated SPE with high sample throughput, which can be applicable on the industrial scale.
References
- Hess, L.M. and Pohl, G. Perspectives of quality care in cancer treatment: a review of the literature. AHDB 2013, 6(6), 321–9.
- Mavroudi, M.; Zarogoulidis, P. et al. Stem cells’ guided gene therapy of cancer: new frontier in personalized and targeted therapy. J. Cancer Res.Ther. 2014, 2, 22–33.
- Berrueta, L.A.; Gallo, B. et al. A review of solid phase extraction: basic principles and new developments. Chromatographia 1995, 40(7/8), 474–83.
- Allanson, J.P.; Biddlecombe, R.A. et al. The use of automated solid phase extraction in the “96 well” format for high throughput bioanalysis using liquid chromatography coupled to tandem mass spectrometry. Rapid Commun. Mass SP 1996, 10, 811–16.
- Marinova, M.; Artusi, C.; et al. Immunosuppressant therapeutic drug monitoring by LC-MS/MS: workflow optimization through automated processing of whole blood samples. Clin. Biochem. 2013, 46, 1723–7.
- Junginger, S. and Thurow, K. Automatisierte festphasenextraktionseinheit mit positivem Druck (automated solid phase extraction unit with positive Druc). Jahresbericht, Nachwuchsgruppe LSA Systems & Process Technologies, 2014, 1–5.
- Elkhalifa, A.S. and Weiner, H.L. Cyclophosphamide treatment of MS: current therapeutic approaches and treatment regimens. The International MS Journal 2010, 17, 12–18.
- Tashkin, D.P.; Elashoff, R. et al. Effects of 1-year treatment with cyclophosphamide on outcomes at 2 years in scleroderma lung disease. Am. J. Resp. Crit. Care 2007, 176, 1026–34.
- Singh, N.; Nigam, M. et al. Caspase mediated enhanced apoptotic action of cyclophosphamide and resveratrol-treated MCF-7 cells. J. Pharmacol. Sci. 2009, 109, 473 – 85.
- Nilsson, K.; Andersson, M. et al. Phospholipid removal combined with a semi-automated 96-well SPE application for determination of budesonide in human plasma with LC-MS/MS. J. Chromatogr. B 2014, 970, 31–5.
- Chen, X.-H.; Franke, J.-P. et al. Pitfalls and solutions in the development of a fully automated solid-phase extraction method for drug screening purposes in plasma and whole blood. J. Anal. Toxicol. 1993, 17, 421–6.
Carolin Gallert, Ellen Vorberg, Thomas Roddelkopf and Kerstin Thurow are with the Center for Life Science Automation (celisca), University of Rostock, Friedrich-Barnewitz Str. 8, D-18119 Rostock, Germany; tel.: +49 381 498 7803; fax: +49 381 498 7802; e-mail: [email protected]. Steffen Junginger and Heidi Fleischer are with the Institute of Automation (IAT), University of Rostock, Rostock, Germany. The authors wish to thank the Federal Ministry of Education and Research (BMBF, Bonn, Germany) for financial support (FKZ: 03Z1KN11), and Mrs. Grit Koch (University of Rostock) for her excellent technical assistance.