The enantiomeric composition of
proline is commonly determined
using conventional analytical
methods such as HPLC, GC,
and CE. Due to the relatively long analysis
times of these methods, high-throughput
requirements often cannot be fulfilled.
A method based on parallel kinetic resolution
and electrospray ionization–mass spectrometry (ESI-MS) with pseudo-enantiomeric
mass-tagged auxiliaries has
been developed that enables enantiomeric
excess determination of proline in approx.
2 min per sample. The electrospray ionization–mass spectrometry method is fully
automated, including sample pretreatment
using liquid handlers and software-based
data processing.
Determination of proline
Chiral amino acids have great impact on
many physiological processes in plants,
animals, and humans. One of the most
important amino acids is proline, since
it is incorporated in many biochemical
pathways and exhibits neurotoxic
effects. In the 1970s, studies were performed
that showed that L-proline caused
amnesia in chicks.1,2 Studies also showed
that D-proline led to convulsions and
death in chicks, although no significant
amnesiac effect was seen.3 More recent
research based on experiments with chicks
showed that L-proline influences the
stress-induced dopamine and serotonin
metabolism,4 and that both enantiomers
can induce sedative and hypnotic effects.5
These applications in the field of neurology
research show the importance of the
determination not only of the total proline
concentration, but also of the differentiation
between the D- and L-enantiomers
and determination of enantiomeric excess.
A number of studies investigated the
determination of D-amino acids in mammals
using HPLC,6–10 GC, CE, and other
analytical techniques.11,12 Most of these
techniques require long analysis times as
well as cost-intensive columns and materials.
In the face of the increasing cost of
today’s health-care systems, the development
of cost-effective, rapid analytical
methods is required to ensure not only cost
reduction but also an increase in the number
of patients who can be screened.
Determination of D-/L-proline
using parallel kinetic
resolution
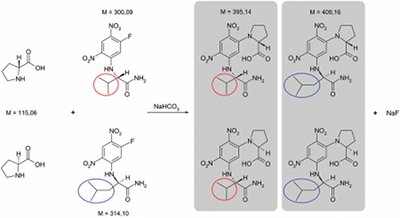
Figure 1 - Reaction equation for derivatization of D- and L-proline.
Mass spectrometry is a fast, reliable
method for the analysis of complex mixtures.
The parallel kinetic resolution
method enables differentiation between
enantiomers using slight differences in
their reaction behavior with other chiral
compounds, and has been used for the
analysis of secondary alcohols, primary
and secondary amines,13,14 carboxylic
acids, amino alcohols, amino acid esters,
and natural compounds.15,16 The chiral
mixture of two enantiomers in a variable
ratio is derivatized with two mass-tagged
pseudoenantiomeric auxiliaries.
A suitable mass difference of the auxiliaries
causes four reaction products with
two characteristic masses. The ratio of
these masses is related to the enantiomeric
ratio of the chiral substrates and
is used for enantiomeric excess calculation.
A classic derivatization agent
used in amino acid analysis is Nα-(2,4-dinitro-5-fluorophenyl)-L-valinamide
(L-FDVA): Marfey’s reagent.17,18 As a
corresponding auxiliary with a mass difference
of 14 and opposed chiral constitution,
Nα-(5-fluoro-2,4-dinitrophenyl)-D-leucinamide (D-FDLA) can be used,
whereby reaction products are formed
with characteristic masses of m/z =
395.14 and m/z = 409.16 (Figure 1).
Experimental
Chemicals and reagents
D- and L-proline (both ≥99%), acetone
(≥99%), and formic acid (~98%) were
obtained from Sigma Aldrich (Steinheim,
Germany). Nα-(2,4-dinitro-5-fluorophenyl)-D-valinamide (D-FDVA)
and Nα-(2,4-dinitro-5-fluorophenyl)-L-valinamide
(L-FDVA) (both ≥98%) were
obtained from Fluka (Buchs, Switzerland).
Nα-(5-fluoro-2,4-dinitrophenyl)-D-leucinamide (D-FDLA) and Nα-(5-fluoro-2,4-dinitrophenyl)-L-leucinamide
(L-FDLA) (both 98%) were obtained from ABCR (Karlsruhe, Germany). Methanol
(HPLC gradient grade) and hydrochloric
acid (37%) were from Roth (Karlsruhe,
Germany), and sodium bicarbonate
(≥99.5%) was from AppliChem (Darmstadt,
Germany).
Sample preparation
A stock solution of each enantiomer (50
mmol/L) was prepared by dissolving the
crystalline proline in 3 mL hydrochloric
acid (1N), neutralizing with 1N sodium
bicarbonate, and filling up to 10 mL with
ultrapure water. For experiments conducted
within the following few days, 2 mL was
filled in separate vials and stored at +2
°C (35.6 °F). The remaining solutions
were frozen at –18 °C (–0.4 °F). Prior to
the derivatization, the stock solution was
diluted (1:50, v/v) to a concentration of 1
mmol/L with ultrapure water. The auxiliary
solution contained L-FDVA and D-FDLA
dissolved in acetone, each with a concentration
of 2.5 mmol/L. For the calibration,
the diluted proline solutions were used to
prepare five mixtures with defined enantiomeric
excesses of +100, +50, 0, –50,
and –100 ee%. The derivatization was performed
in 1-mL GC vials with screw caps
(Agilent Technologies, Waldbronn, Germany)
as well as in 96-well master blocks
with a well volume of 500 μL (Greiner-BioOne, Essen, Germany). Fifty microliters
of the chiral substrates, 100 μL of the
auxiliary solution, and 20 μL of sodium
bicarbonate (1 M) were added and mixed
in a thermo shaker (Thermomixer comfort,
Eppendorf, Hamburg, Germany) at
750 rpm for 1 hr at 20 °C. Subsequently,
10 μL hydrochloric acid (2 M) was added
and the samples were mixed for 5 min more.
Finally, 320 μL of methanol was added and
the samples were mixed for a few seconds.
Instrumentation and analysis
parameters
The mass spectrometric analyses were carried
out on an Agilent LC-MS system
with the following units: G1379B vacuum degasser, G1312B binary pump, G1367C
high-performance
automated liquid sampler,
and G1969A time-of-flight mass spectrometer (TOF-MS) with an electrospray
ion source (electrospray ionization, ESI).
Five microliters of the sample solution was
injected with prior needle wash. A methanol–water mixture (90:10, v/v) containing
0.1% formic acid was used as mobile phase
for the sample injection at a flow of 0.35 mL/min. The TOF-MS was operated in negative
ion mode with the following parameters:
nitrogen as nebulizer and drying gas, 35
psig nebulizer pressure, 10 L/min drying gas
flow, 300 °C drying gas temperature, 4000 V
capillary voltage, 215 V fragmentor voltage,
60 V skimmer voltage, and 250 V octupole
voltage. Data acquisition, extraction of the
peak areas of the required masses, and integration
of these peak areas were performed
using MassHunter Data Acquisition and
MassHunter Qualification software (Agilent
Technologies). Enantiomeric excess calculation
and visualization of the results were
realized with the software module “Chiral
MS” self-implemented for these special tasks.
This module was implemented using Microsoft
Office Excel 2007 with Visual Basic 6.5
(Microsoft Corp., Redmond, WA).
Results and discussion
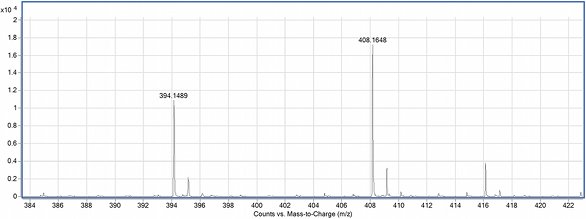
Figure 2 - Mass spectrum of the derivatives of a proline racemate.
The ratio of the characteristic m/z values
of the derivatives was used for subsequent
enantiomeric excess determination. The
method was tested under various conditions
during the sample preparation. Furthermore,
a number of validation procedures were performed,
and finally compared with a conventional
analysis technique. Figure 2 shows a
mass spectrum with the characteristic masses
m/z = 394.14 and 408.16 of the detected
[M–H]– ions for a proline racemate.