Food safety detection requirements demand rapid analytical methods in both the laboratory and field. High-performance ion mobility spectrometry (HPIMS) provides the speed and portability of previous IMS benchtop instruments, but with resolving power comparable to liquid chromatography (LC). Direct electrospray ionization (ESI) from a sample syringe adds the advantages of ESI to detect priority targets such as drug residues, dyes, and plasticizers, even in food matrices, for potential on-site screening with higher throughput.
Introduction
Consumers rely on screening protocols to ensure the safety of the food supply reaching the market. Identifying chemical contaminants present becomes the detection challenge, whether the adulteration occurs accidentally during the growing and/or production process, or intentionally for enhancing profit.1,2 Ideally, screening methods should be sensitive, selective, and portable, allowing the technique to be used where contamination could be introduced.
For these reasons, ion mobility spectrometry applications for analysis in the burgeoning foodomics field are expanding.3 IMS is a widely adopted gas–phase sample separation and detection technique ubiquitous to security screening in aviation and law enforcement to find trace amounts of explosives and drugs of abuse in the field. IMS also has a long history of chemical weapons agent (CWA) monitoring by the military.4,5 These instruments are compact and utilize primarily room air as the separating medium with total analysis times in seconds. However, the systems also suffer from low resolution.
Currently, many food screening protocols utilize gas or liquid chromatography (GC or LC) to detect the target constituents, methods which are not as easily adapted to on-site or field use. Subsequently, many previous IMS applications have focused on detecting emitted volatiles associated with food and beverage contamination via atmospheric pressure chemical ionization methods (APCI) for sample introduction.3,6 However, electrospray ionization sources are being implemented more.7–10 To address unmet needs in food safety aimed at faster, potentially more portable screening methods, the current work utilizes the GA2100 ESI-HPIMS (Excellims Corp., Acton, MA) to demonstrate rapid detection of several high-priority contaminants including phthalates, consumer-grade dyes, and veterinary drug residues. This work expands upon earlier studies using ESI-HPIMS for analyzing nutritional supplements7 and proof-of-concept food safety studies.11
High-performance ion mobility spectrometry with electrospray ionization
High-performance ion mobility spectrometry has two key components: 1) a source for introducing and ionizing samples, and 2) the HPIMS analyzer for separating them. Combining an ESI source with HPIMS provides a powerful combination where substances that can be dissolved in solvent or are already liquids can be readily analyzed. ESI is well-known as a “softer” ionization source preserving the native structure of the analyte and minimizing fragmentation before entering the instrument.7,11–13
For the current work, the GA2100 standalone benchtop ESI-HPIMS shown in Figure 1 (right) has been used with the Directspray ESI source (Excellims). Directspray ionizes the sample by ESI directly from the 100-μL gas-tight syringe needle containing the sample. This syringe can simply be rinsed 2–3 times prior to analyzing another sample, eliminating the long capillary tubing of traditional infusion-type ESI sources and minimizing carryover.
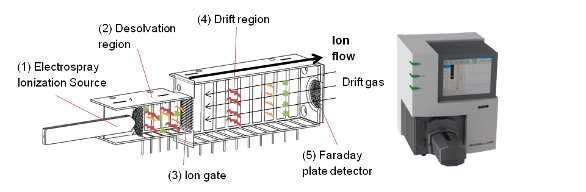
Figure 1 – High-performance ion mobility spectrometry (HPIMS) instrument (left); GA2100 ESI-HPIMS with Directspray ESI source (right).
The instrument in Figure 1 (left) operates at atmospheric pressure, eliminating vacuum pumps needed for low pressures, and uses air as the mobility drift medium. The ions from the ESI source (1) have remaining ESI solvent removed in the desolvation region (2) prior to injection into the drift region using a Bradbury-Nielsen (BN) gate (3). After the mobility-based separation in the drift region (4), the ions are detected at a Faraday plate detector (5). Table 1 provides the HPIMS instrument settings used for the experiments discussed.
Figure 1 conceptually demonstrates HPIMS. The gas phase ions of a sample’s components are separated in the drift region (4) based on their differences in structural size and shape that cause them to “move” differently based on collision interactions with a counter-flowing neutral drift gas as an electric field guides them. Smaller, more compact ions (green shapes) arrive at the detector before larger, bulkier ones do (red and orange shapes), analogous to time-of-flight (TOF) methods. This separation mechanism is orthogonal to both mass spectrometry (MS) separation based on mass-to-charge ratio (m/z), and LC, which separates by polarity. Consequently, HPIMS gives valuable information about the molecules in a sample in a very short time, requiring only milliseconds for ions to travel the length of the drift tube, with complete averaged spectra obtained in under 60 sec.
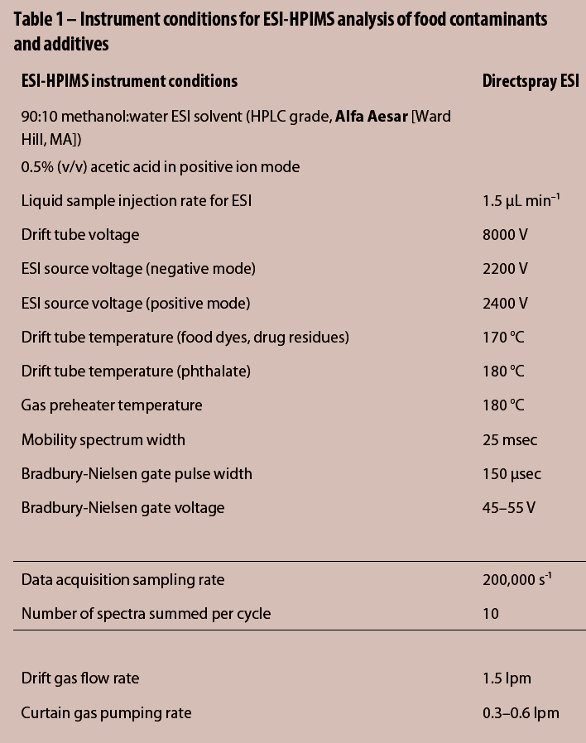
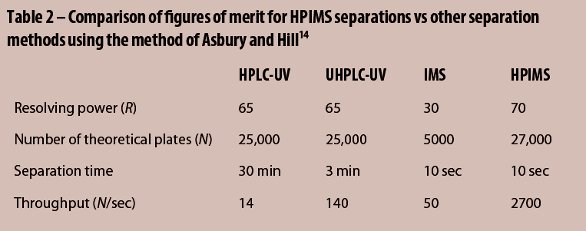
To achieve its performance metrics in Table 2,14 HPIMS leverages advances in ion mobility instrument construction to provide the speed and simplicity of IMS with figures-of-merit comparable to high-performance and ultrahigh-performance liquid chromatography (HPLC and UHPLC) methods traditionally used in food screening. The resolving power, R, for IMS can be defined as the ratio of the drift time, td, to the peak width at half-maximum, wh = Δ1/2td.4,14 HPIMS routinely gives R of 60 to 70, comparable to HPLC and UHPLC methods. Similarly, the number of theoretical plates (N) for HPIMS using the ratio14 5.55(td/wh)2 is up to 27,000, again very similar to the two LC methods. However, the HPIMS analysis typically completes in as little as 10 sec, giving almost 200-fold improvement in throughput (N/sec) vs LC.
ESI-HPIMS detection of phthalates, additives, and residues
Phthalate plasticizers are commonly used in the manufacture of everyday items such as personal care products (PCPs), toys, and food packaging.15 Concern over frequent, long-term exposure to these compounds is mounting16 because of evidence supporting their role as endocrine disruptors that increase developmental abnormalities and impair reproduction and development.17–19 Phthalates have further been used as illicit clouding agents in food and drinks produced outside of the United States.20 Therefore, detection of phthalates in beverages has been demonstrated using ESI-HPIMS.11
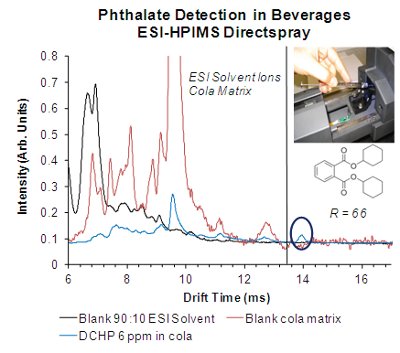
Figure 2 – ESI-HPIMS mobility spectra for detecting dicyclohexyl phthalate (DCHP) in cola using Directspray ESI in positive ion mode.
Previous proof-of-concept experiments show that ESI-HPIMS is adept at detecting the main phthalate compounds banned internationally, even in food matrices.11 An array of 12 phthalates could be detected to ppb levels with a 1.5 order-of-magnitude linear response range, producing [M+nH]n+ and [M+Na]+ peaks. The previous work has used a traditional infusion-type ESI source, requiring long times to clean the capillary lines between analyses.11 Therefore, Directspray ESI has alternatively been used to detect dicyclohexyl phthalate (DCHP, ULTRA Scientific, North Kingstown, RI) in cola for faster sample analysis.
First, a blank 90:10 methanol:water ESI solvent was analyzed with the Directspray ESI-HPIMS in positive ion mode. Next, 100 μL of off-the-shelf cola was diluted with 900 μL of methanol, and the mobility spectrum for the blank cola matrix was measured. The same dilution with another 100 μL of cola was prepared, but this sample was spiked with 6 ppm of DCHP. As clearly seen in Figure 2, a distinct DCHP peak can be detected using Directspray ESI and HPIMS with resolving power of 66 at 6 ppm, a level 25 times below the detection limits of the Chinese liquid chromatography method for phthalate mixtures.21,22 However, the data acquisition only required 30 sec with HPIMS. Furthermore, only three rinses of the syringe between the samples were used, increasing the method throughput.
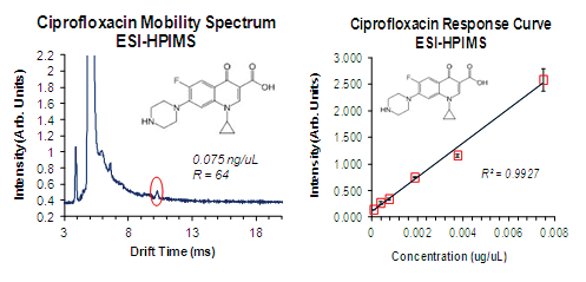
Figure 3 – ESI-HPIMS mobility spectrum for 0.075 ng μL–1 (75 μg L–1) of ciprofloxacin in positive ion mode (left); response curve for ciprofl oxacin using positive ion mode ESI-HPIMS (right).
Another area of concern is overexposure to antibiotics that can lead to resistance to their therapeutic effects.23 The prevalent use of antibiotics in the livestock industry for infection prevention has prompted the legislation of residue limits for veterinary drugs in muscle tissue for consumption.24Figure 3 (left) shows the spectrum for 0.075 ng μL–1 (75 μg L–1) of a ciprofloxacin standard (Sigma Aldrich, St. Louis, MO), a fluoroquinolone antibiotic and metabolite of enrofloxacin,24 measured with ESI-HPIMS in positive ion mode with resolving power of 64 in 30 sec without sample preconcentration. Moreover, the linear response range for ciprofloxacin covers two orders of magnitude vs sample concentration with excellent precision.
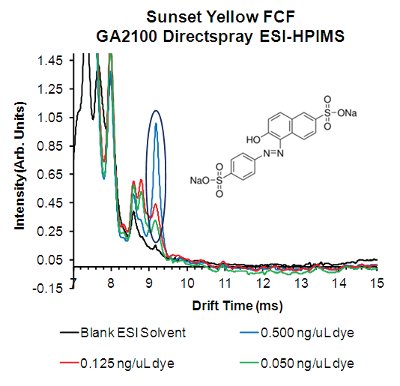
Figure 4 – ESI-HPIMS mobility spectra for Sunset Yellow FCF food dye using Directspray ionization in negative ion mode.
Another prime detection target is additives such as food dyes, both legal and illegal. In addition to detecting industrial dyes illicitly added to inexpensively enhance food color,25 the legal azo-based food grade dyes (e.g., FD&C artificial colorants) have been cited as potential triggers for food sensitivities. 26 ESI-HPIMS has previously shown excellent response for detecting the azo-based artificial food colorings and controlled artificial sweeteners.11Figure 4 shows ESI-HPIMS mobility spectra in negative ion mode for Sunset Yellow FCF (FD&C Yellow 6). A distinct peak for the dye anion at concentrations as low as 0.050 ng μL–1 (~60 ppb) can be seen in Figure 4 (blue curve) from a 30-sec acquisition, compared to the over 6-min elution time with HPLC.27 Previous ESI-HPIMS studies have shown that the mobility peaks correspond to the dye anion from the commonly produced azo dye-salts. These dyes can also be detected with two-order-of-magnitude linear response range in concentration.11
Conclusion
ESI-HPIMS has been shown to be a sensitive and selective method for analyzing compounds relevant to current food safety priorities. Separating power comparable to HPLC and UHPLC can be achieved in seconds with the added benefit of being able to directly ionize samples from the syringe used for injection via Directspray ESI, affording greater throughput in sample processing and creation of “dilute-and-shoot” methods. This simplifi ed sample preparation and direct analysis has been demonstrated for detecting phthalates in beverages with good sensitivity.
References
- Dorne, J.L.C.M.; Bordajandi, L.R. et al. TrAC Trends Anal. Chem. 2009, 28, 695.
- Arvanistoyannis, I.S. In: Food Contaminants and Residue Analysis; Pico, Y., Ed.; Elsevier: Amsterdam, The Netherlands, 2008; Vol. 51, p 29.
- Karpas, Z. Food Res. Int. 2013; in press.
- Eiceman, G.A.; Karpas, Z. Ion Mobility Spectrometry; 2nd ed.; CRC Press: Boca Raton, FL, 2005.
- Keller, T.; Keller, A. et al. For. Sci. Int. 2006, 161, 130.
- Karpas, Z.; Guaman, A.V. et al. Anal. Chim. Acta 2013, 758, 122.
- Krueger, C.A.; Midey, A.J. et al. Int. J. Ion Mobil. Spectrom. 2011, 14, 71.
- Jafari, M.T.; Rezaei, B. et al. Food Chem. 2011, 126, 1964.
- Jafari, M.T.; Torki, F. et al. Anal. Sci. 2012, 28, 391.
- Fernandez-Maestre, R.; Hill, H.H. Int. J. Ion Mobil. Spectrom. 2009, 12, 91.
- Midey, A.J.; Camacho, A. et al. Anal. Chem. 2013; submitted.
- Hilton, C.K.; Krueger, C.A. et al. Int. J. Mass Spectrom. 2010, 298, 64.
- Krueger, C.A.; Hilton, C.K. et al. Int. J. Ion Mobil. Spectrom. 2009, 12, 33.
- Asbury, G.R.; Hill, Jr., H.H. J. Microcolumn Sep. 2000, 12, 172.
- Staples, C.A., Ed. Phthalate Esters; Springer: New York, 2003.
- Trasande, L.; Attina, T.M. et al. Environ. Health Persp. 2013; in press.
- Heudorf, U.; Mersch-Sundermann, V. et al. Int. J. Hyg. Envir. Heal. 2007, 210, 623.
- Rudel, R.A.; Camann, D.E. et al. Environ. Sci. Technol. 2003, 37, 4543.
- Fourth National Report on Human Exposure to Environmental Chemicals; Department of Health and Human Services. Centers for Disease Control and Prevention, 2009.
- U.S. FDA Notification to Industry Regarding Potential Adulteration of Food Products used as Emulsifying/Clouding Agents and Flavorings; Food and Drug Administration, 2011.
- GB/T 21911-2008. Food Determination of Phthalates; Standardization Administration of China, 2008.
- Phthalates Determination Method with Liquid Chromatography for Twelve Phthalates in Machinery and Electronics Products; National Certification and Accreditation Administration Committee, Plan Item No. 2009B463.2k, 2009.
- Waters, A.E.; Contente-Cuomo, T. et al. Clin. Infect Dis. 2011, 10.1093/ cid/cir181.
- Official J. Eur. Union 2010, L15, 1.
- Soylak, M.; Unsal, Y.E. et al. Food Chem. Toxicol. 2011, 49, 1796.
- Food Allergy: Adverse Reactions to Food and Food Additives, 4th ed.; Metcalfe, D.D., Ed.; C.H.I.P.S.: Weimar, TX, 2008.
- Zou, T.; He, P. et al. Food Chem. 2013, 138, 1742.
Anthony J. Midey, Ph.D., is Senior Scientist/Project Manager; Clinton A. Krueger, Ph.D., is Applications Development Lead; Mark A. Osgood, M.Sc., is Vice President Engineering; Jianglin Wu, Ph.D., is Senior Software Engineer; and Ching Wu, Ph.D., is President and CEO, Excellims Corp., 20 Main St., Acton, MA 01720, U.S.A.; tel.: 978-264-1980; fax: 978-264-1981; e-mail: [email protected]; www.excellims.com.