One of the most valuable emerging tools for prognostic indication of cancer is the detection and quantification of circulating tumor cells (CTCs). However, the full potential of this tool in personalized therapy and translational research is bound to the molecular profiling of CTCs. Enrichment techniques currently available provide a level of purity incompatible with the majority of molecular analyses. CTC technology uses image-based single-cell sorting from small cell loads and is designed to deliver 100% pure cells which, following whole genome amplification, may provide the key to the unraveling of their molecular profile.
Clinical background
Patients with various metastatic carcinomas over a wide range of frequencies have been found to have regularly circulating tumor cells.1,2 These CTCs are extremely rare in healthy individuals and patients with nonmalignant diseases. Some clinical studies indicate that the assessment of CTCs may assist physicians in monitoring and predicting cancer progression and in evaluating response to therapy in patients with metastatic cancer.3–5
In patients diagnosed with metastatic breast cancer, the number of CTCs has been shown to be a significant predictor of progression-free survival (PFS) and overall survival (OS).6,7 In a study by Cristofanilli et al.,7 patients undergoing treatment for metastatic breast cancer with >5 CTCs/7.5 mL whole blood had shorter median PFS (2.7 months vs 7.0 months, P<0.001) and shorter OS (10.1 months vs >18 months, P<0.001) than patients having <5 CTCs/7.5 mL blood. In the same study, a decrease in the number of CTCs to <5 from baseline to first follow-up (4–5 weeks after initiation of new therapy) was also found to be predictive of PFS and OS. These results suggest that the number of CTCs is a useful prognostic guide for patients with metastatic breast cancer and can reliably estimate disease progression and survival earlier (4–5 weeks vs 8–12 weeks, respectively) than traditional imaging methods.
Individual cell detection and recovery
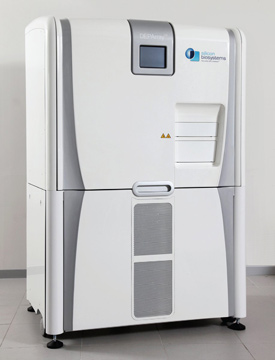
Figure 1 - DEPArray system.
The DEPArray™ system (Silicon Biosystems, Bologna, Italy) (Figure 1) is a cell-trapping microarray of movable cages of electrical potential that implements a patented technology for individual rare cell detection and recovery from suspensions. The system is driven by a microelectronic active silicon-substrate-embedding control circuitry that addresses each individual dielectrophoretic (DEP) cage. This results in high flexibility and selectivity and is a significant development in biological research and analysis.
The tiny electrodes in the chip permit the DEP cage sizes to accommodate as little as one single cell, enabling parallel individual manipulation of up to 100,000 cells. The device embeds more than 300,000 20 μm × 20 μm electrodes on a chip surface, which allows the creation of up to 76,800 DEP cages between the chip surface and a single electrode transparent lid. The lid is placed 90 μm above the chip surface and forms the manipulation and separation chamber. The DEP cages permit cell capture and motion. However, identification and selection of the cells is accomplished through a fluorescence microscope to obtain a multiparametric image-based selection, thus allowing recovery of 100% pure cells. An important aspect of the system is the absence of shear force stress on cells during manipulation. Clear evidence has been shown that cells recovered in this way maintain their viability; their DNA is completely intact, and their proliferation capability is unmodified. This transversal technology offers different possible applications such as prenatal and cancer (following pure CTC recovery) diagnosis, cell therapy, and single-cell biology.
The benefits of the DEPArray system in comparison to available fluorescence-activated cell sorting (FACS) include:
- The system can sort specific cells that are extremely rare in the original suspension, based on relative fluorescence parameters combined with morphology
- Cells can be sorted from a suspension in which the total cell count is extremely low
- A priori thresholds are not needed; only the best available cells in the sample are picked up
- There is no contamination from spurious events, verifying images associated to cells selected from the scatter plot or histogram, including fluorescence and brightfield
- The system has the ability to sort by morphological parameters such as shape, nucleus-to-cytoplasm ratio, fluorophores co-localization, due to image-based selection
- Slow kinetics events can be detected.
Applications
The method of sorting used in the DEPArray system bridges the gap between enrichment techniques and single-cell molecular analysis methods and delivers very high purity for rare cell analysis. Beyond sorting, the cell handling capability of the system allows researchers to carry out digitally controlled experiments that are not possible with existing techniques, i.e.:
- Rare-cell sorting: Isolation of pure CTCs, or fetal cells circulating in maternal blood, with image-based selection
- Cell sorting of small cell loads: Starting sample may range from tens to tens of thousands of cells
- Investigation of cell–cell interaction, e.g., the study of cell lysis mechanism induced by cytotoxic cells
- Single-cell targeting: By using functionalized microbeads and target cells, it is possible to stimulate cell surface receptors in a controlled way
- Drug delivery: May deliver tiny amounts of compounds into the cell using proper carriers.
Additional features
The DEPArray system uses a highly automated process, allowing individual cell operation with high selectivity and flexibility. The analytical protocol can be digitally controlled by a computer program, enabling straightforward automation and customization. Because the cells are manipulated by levitation inside the microchamber, they cannot stick to the device surfaces. Since the no-channel architecture does not rely on capillaries or micromachined channels, the risk of clogging is reduced. Even if clogs occur, cells can be routed easily around the obstacles.
References
- Molnar, B.; Sipos, F. et al. Molecular detection of circulating cancer cells. Dig. Dis. 2003, 21, 320–5.
- Allard, W.J.; Matera, J. et al. Tumor cells circulate in the peripheral blood of all major carcinomas but not in healthy subjects or patients with nonmalignant diseases. Clin. Cancer Res. 2004, 10, 6897–6904.
- Aquino, A.; Prete, S.P. et al. A novel method for monitoring response to chemotherapy based on the detection of circulating tumor cells: a case report. J. Chemother. 2002, 14, 412–16.
- Katoh, M.; Neumaier, M. et al. Correlation of circulating tumor cells with tumor size and metastatic load in a spontaneous lung metastasis model. Anticancer Res. 2004, 24, 1421–5.
- Berrepoot, L.V.; Mehra, N. et al. Increased levels of viable circulating endothelial cells are an indicator of progressive disease in cancer patients. Ann. Oncol. 2004, 15, 139–45.
- Weigelt, B.; Bosma, A.J. et al. Marker genes for circulating tumour cells predict survival in metastasized breast cancer patients. Br. J. Cancer 2003, 88, 1091–4.
- Cristofanilli, M.; Budd, T. et al. Circulating tumor cells, disease progression, and survival in metastatic breast cancer. N. Engl. J. Med. 2004, 351, 781–91.
Mr. Gannon is a Communications Consultant, Au Pied du Chateau, Rue Guillaume Taffanel, 34210-Cesseras, France; tel.: +33 (0) 4 68 43 82 58; e-mail: [email protected].