The basis for the physical properties of a (polymeric) material is found in its chemical composition. If one can properly determine the polymer’s composition, one will gain a better understanding of the polymer and the ability to predict its physical behavior. Especially for synthetic polymers containing more than one monomer, such as copolymers, the composition usually becomes complex and is not as easy to determine. Liquid chromatography coupled with mass spectrometry (LC-MS) is a technique that has become very important in many fields of analytical chemistry in the last decade. After a somewhat delayed start, LC-MS is also becoming more widely used in synthetic polymer characterization,1,2 as demonstrated in this article.
Liquid chromatography can be used in different forms. The most widely used technique for synthetic polymers is size exclusion chromatography (SEC), although it only yields information on the molecular weight of the polymer and how the molecular weight is distributed (MWD).1,3 More information on the chemical composition of the polymer is obtained by liquid chromatography at critical conditions (LCCC), where separation is based on the number of one comonomer and is independent of the other comonomer. 1,3 Also, conventional (reversed-phase) liquid chromatography (RPLC) is suitable for obtaining chemical information, since the complete chemical composition plays a role in the separation. 1,3 Even higher chromatographic resolution can be obtained with two-dimensional chromatographic techniques, 2 which are particularly useful for more complex samples.
In the MS analysis of synthetic polymers,1,4,5 matrix-assisted laser desorption/ionization (MALDI) is the most widely used ionization technique, followed by the slightly softer method of electrospray ionization (ESI), which is commonly used for the analysis of biomolecular species.1,2 Nevertheless, ESI has the advantage of multiple charging1 (therewith extending the applicable polymer mass range) and benefits from less ionization-induced fragmentation.4 Furthermore, ESI-MS is suitable for on-line LC coupling, which is not as common for MALDI-MS.1–5Atmospheric pressure chemical ionization (APCI), which is recognized as the better choice for ionizing less polar species and is also well suited for LC-MS, is not often used as an ionization technique for synthetic polymers. APCI has a limited accessible mass range and is therefore not appropriate for high-molecular-weight polymers. However, since not all polymer species are as easily ionized by (positive ion) ESI, its application in addition to ESI can provide valuable data in the analysis of low-molecular-weight polymeric samples. This is demonstrated in the current example, which describes the characterization of synthetic block copolymeric surfactants based on methyl poly(ethylene oxide) (mPEO) and e-caprolactone (CL) and functionalized with linoleic acid (LA) by means of RPLC-ESI-MS and RPLC-APCI-MS, using ionization in both the positive and negative ion modes.
Experimental
All analyses were performed using an HP1100 liquid chromatograph (Agilent Technologies, Waldbronn, Germany) coupled to an esquire 3000plus ion trap mass spectrometer (Bruker Daltonics, Bremen, Germany). Reversed-phase chromatographic separation was performed on a 2.1 × 150 mm C-8 column (Macherey-Nagel, Düren, Germany) with the following gradient of acetonitrile (solvent B) in 20 mM aqueous ammonium formate buffer (pH ~3, solvent A) at a flow rate of 300 μL/min: 2 min isocratic at 10% B (v/v), increase of solvent B to 95% over 38 min, 95% B for 39 min, return to 10% B within 1 min, and equilibrate the column at those conditions for 10 min. Injection volumes of sample or blank solutions were 5 μL. Both ESI and APCI were employed for ionization and these were operated in the positive and negative ion modes. The mass spectrometer was operated in full-scan mode, recording mass spectra in the range of m/z 150–3000 with the target mass set to m/z 1500. All measurements were carried out in duplicate.
Results and discussion
Complexity of the surfactant
Since few reactions have 100% turnover and proceed without side reactions, the respective end product is likely to contain some of the starting material as well as side products, and further cleanup is required to obtain a pure product. For block copolymers, side reactions may include homopolymerization for each of the comonomers and various intermolecular reactions. When a follow-up reaction, e.g., functionalization, is performed, functionalization of the side products may occur as well and incomplete functionalization will further expand the product’s complexity. Purification steps may be incorporated after the first and second reaction steps, but are often not fully successful. Thus, for the given block copolymer, unreacted poly(ethylene glycol) (mPEG), homopolymer of caprolactone (pCL), block copolymer (mPEGCLn), and linoleic acid derivatives of these (xxx-LA) may be found in the sample. In addition, each product is a polymer and exhibits a molecular weight distribution (homologous series), thus completing the “polymeric chaos.” It is this complexity that we want to separate and identify.
Separation
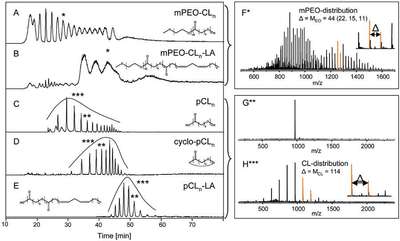
Figure 1 - LC-MS chromatograms showing the separation of the homologous polymer series contained in the block copolymer samples. a) Total ion chromatogram (TIC) of unfunctionalized polymer, recorded with ESI(+)-MS. b) TIC of functionalized polymer, recorded with ESI(+)- MS. c) Multiple ion chromatogram (MIC) of 17 selected mass traces of unfunctionalized polymer, recorded with APCI(–)-MS. d) MIC of 14 selected mass traces of unfunctionalized polymer, recorded with APCI(+)-MS. e) MIC of 13 selected mass traces of functionalized polymer, recorded with APCI(+)-MS. Typical mass spectra are given for the marked peaks, as well as for the complete homologous series shown in F, G, and H. (Figures 1a–e reproduced with permission from Ref. 6.)
The chromatographic separation obtained for the different products and their inherited homologues is depicted in Figure 1. The individual homologous series are (partially) separated, and within the products CL homologues are separated. EO homologues are separated only to a minor extent, resulting in relatively broad peaks containing the complete mPEO distribution. The incomplete separation of the different products (individual homologous series) could lead to loss of information and thus incomplete characterization, but this is overcome by the use of an appropriate detection system (see below). In the current separation system, polymer species that are too hydrophobic will not or will only gradually elute from the column (yielding broad peaks; see Figure 1b) and information may be lost as well. However, selecting a different or further optimized chromatographic system may solve this problem.
Identification
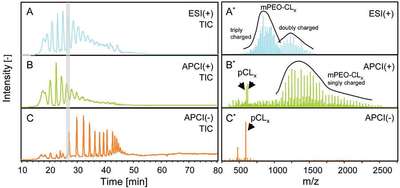
Figure 2 - LC-MS chromatograms (TICs) of nonfunctionalized copolymer, based on nonpurified starting materials (left) and the respective mass spectra for the highlighted time frame (right). Results obtained with a) positive ESI, showing (mainly) the separated copolymer products; b) positive APCI, showing both copolymer and homopolymer in the spectrum; and c) negative ion APCI, showing predominantly the separated homopolymer products. (Figure 2 reproduced with permission from Ref. 6.)
Detection based on different ionization systems [ESI(+), APCI(+), and APCI(–)] resulted in completely different chromatograms for the same polymer sample (Figure 2), which is especially the case for LC-MS runs with ESI(+) and APCI(–). This points out that with different ionization methods, different polymeric species are visualized or emphasized. For the respective block copolymer, positive ion electrospray was found to favor the ionization of all mPEO-containing species, whereas the side products without the mPEO chain are barely observed. Negative ion APCI shows the opposite: favored ionization of the (linear) CL homopolymer and its LA derivative, against low intensities (if at all) for mPEO-containing species. On first sight, ionization with APCI(+) seems to be similar to ionization with ESI(+), and one may conclude from the corresponding mass spectrum (Figure 2b) that APCI(+) more or less combines the ionization power of ESI(+) and APCI(–). However, the sensitivity for the distinct species in ESI(+) and APCI(–) is much higher than in APCI(+), and the most complete polymer profile is obtained using the three methods in parallel for the mentioned species [using APCI(+) for the cyclic polycaprolactone]. The only ionization method that does not provide additional information for this surfactant is ESI(–). Further details on the use of the different ionization methods are described elsewhere.6
Characterization of different surfactants
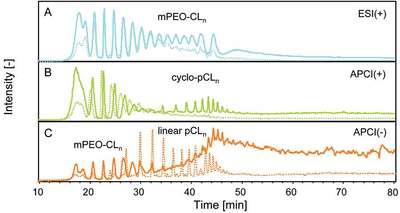
Figure 3 - LC-MS chromatograms (TICs) of nonfunctionalized copolymer, based on nonpurified starting materials (dotted line) and dried starting materials (solid line), recorded with a) ESI(+), showing an increase of high-n block coplymer; b) APCI(+), showing a strong increase of cyclic polycaprolactone; and c) APCI(–), showing a strong decrease of linear polycaprolactone.
The above-described LC-MS method using different ionization methods in parallel is well suited for semiquantitative analysis, comparing the relative peak intensities of different samples. This was used to investigate the influence of the purity of the starting materials and the reaction environment on the polymerization and to monitor the reaction progress. Two surfactants were compared: one based on dried starting materials and polymerized under inert conditions, and the other based on nonpurified starting materials and polymerized under normal laboratory conditions. The overall mass distribution for the species is found to be similar in the different surfactants, but the ratio of distinct polymeric species is different, as shown in Figure 3 for the nonfunctionalized copolymer. Surfactant based on purified mPEG and CL is found to contain higher relative amounts of high-n CL block (Figure 3a) and LA functionalized block copolymer. Transesterification of pCLn seems to be favored as well under the given reaction conditions (Figure 3b). The surfactant polymerized under normal laboratory conditions is found to contain larger amounts of linear homopolycaprolactone (Figure 3c) and the LA functionalized homopolymer.
Reaction monitoring
Similarly, samples taken at different times during the course of the reaction were compared and the reaction progress was investigated. In the copolymerization step, low-molecular-weight pCLn is formed quickly, and the number of CL units in the homopolymer increases with increased reaction times, as expected. Further, the formation of cyclic pCLn turned out to require longer reaction times. The LA functionalization was found to occur over the complete pCLn range simultaneously. There is no indication for longer pCLn chains to react slower (or faster) with the fatty acid than the shorter ones since the pattern of homologues appears similar throughout the reaction.
Conclusion
In the LC-MS analysis of synthetic polymers, application of different ionization methods has proven to add important information to the data commonly obtained by one single method [mostly ESI(+)]. Due to diverse polarities of products contained in complex synthetic (co)polymers (which become complex as a result of incomplete and impure reactions and difficult cleanup), one specific ionization method will emphasize certain products and overshadow others. As a result, only the parallel use of ESI(+), APCI(–), and APCI(+) allowed the characterization of LA functionalized surfactants based on block copolymerization of mPEO and CL, and a combination of ionization methods is promising in the characterization of other (block) copolymers as well.
References
- Hanton, S.D. Chem. Rev.2001, 101, 527.
- Murgasova, R.; Hercules, D.M. Anal. Chem.2003, 75, 3744.
- Montaudo, M.S. Mass Spectrom. Rev.2002, 21, 108.
- Philipsen, H.J.A. J. Chromatogr. A2004, 1037, 329.
- Pasch, H. Phys. Chem. Chem. Phys.1999, 1, 3879.
- van Leeuwen, S.M.; Tan, B.H.; Grijpma, D.W.; Feijen, J.; Karst, U. Rapid Commun. Mass Spectrom.2007, 21, 2629.
Ms. van Leeuwen and Prof. Dr. Karst are with the Chemical Analysis Group, University of Twente, Enschede, The Netherlands and with the Institute of Inorganic and Analytical Chemistry, University of Münster, Münster, Germany; tel.: +49 251 83 36658; fax: +49 251 83 36013; e-mail: [email protected]. Dr. Tan, Dr. Grijpma, and Prof. Dr. Feijen are with the Department of Polymer Chemistry and Biomaterials, University of Twente.