The quantitative measurement of oxygenated compounds such as alcohols and ethers in gasoline by gas chromatography is difficult, because a single column for the complete separation of the target compounds from the complex hydrocarbon matrix is unavailable. Multidimensional gas chromatography (MDGC) can solve this problem. With MDGC, two columns with different separation characteristics are used. The two columns are connected by a switching device that transfers the unresolved fraction eluting from the first column to the second column, where complete separation can be achieved.
For this article, the authors examined the effectiveness of the technique for the simultaneous analysis of 13 oxygenated compounds in gasoline described by ASTM D4815-99.1
Instrumentation
Measurements were made using an MDGC/GCMS-2010 multidimensional gas chromatograph (Shimadzu Corp., Kanagawa, Japan). The system consists of two GC-2010 gas chromatographs (referred to as the first GC and the second GC) and an AOC-20i autoinjector (Shimadzu). Both GCs feature a split/ splitless injector and a flame ionization detector (FID); however, the split/splitless injector in the second GC was not used in this study. The switching device is driven by a high-precision digital pressure controller (Advanced Pressure Controller, APC), which controls the gas (helium) that is used for pressure switching.
Multidimensional heart-cutting of partial or unseparated peaks was first proposed by Deans in 1965.2 These early Deans switching schemes suffered from shifting retention times as a result of flow disruption in the first column during the heart-cutting process. Advances in GC technology (digital flow and pressure control, precision machining, and incorporation of digital CPU control) have made it possible to build an automated capillary column-based switching unit that allows for multiple heart-cuts in a single run without retention time disruption. The obvious advantage of the system is a heart-cutting method that can be created easily, regardless of the duration of the heart-cut or the number of times it is performed.
The multi-Deans switching mechanism in the MDGC/GCMS-2010 is designed with a low dead volume, resulting in sharp peaks. The internal surface of the switching device has been deactivated, allowing active compounds to move through the switch without disruption of peak shape. For these reasons, higher peak reproducibility is obtained, and analysis with a high level of quantitative accuracy is possible.
Operational conditions
In this study, the oxygenated compounds that were completely separated from the complex hydrocarbon matrix by the first column were measured by the first FID; the unseparated compounds were transferred to the second column and measured by the second FID. Thus, all the target compounds were completely separated and simultaneously measured.
The operational conditions were as follows:
- First GC. Rtx-1 (100% polydimethylsiloxane), 60 m × 0.25 mm i.d., df = 1.0 μm (Restek Corp., Bellefonte, PA), was used as the first column. Carrier gas: He (constant pressure); injection mode: split (1:300); inlet head pressure: 289.1 kPa; inlet temperature: 250 °C; injection volume: 0.3 μL; first column temperature program: 47 °C (hold 16 min), ramp at 30 °C/min to 200 °C, 15 °C/min to 250° C (hold 5 min); APC unit pressure: 100 kPa; switching time: 4.39~4.60 min, 8.18~8.34 min, 9.20~9.35 min, 9.79~9.98 min, and 10.71~10.96 min; first FID temperature: 250 °C.
- Second GC. Rtx-1701 (14% cyanopropylphenyl + 86% polydimethylsiloxane), 30 m × 0.32 mm i.d., df = 1.0 μm (Restek), was used as the second column. Second column temperature program: 35 °C (hold 14 min), ramp at 20 °C/min to 200 °C, 15 °C/ min to 240 °C (hold 5 min); second FID temperature: 250 °C. All data were collected using MDGCsolution software (Shimadzu).
Sample and standard compounds
Regular gasoline was purchased from a local gas station in Kanagawa, Japan. Methyl tert-butylether (MTBE), ethyl tert-butylether (ETBE), tert-amylmethylether (TAME), diisopropylether (DIPE), methanol (MeOH), ethanol (EtOH), isopropanol (i-PrOH) , n- propanol (n-PrOH) , isobutanol (i-BuOH), tert-butanol (t-BuOH), sec-butanol (s-BuOH), n-butanol (n-BuOH), and tert-pentanol (t-AmOH) were purchased from Wako Pure Chemical Industries, Ltd. (Osaka, Japan).
Results and discussion
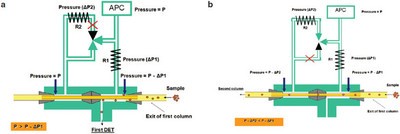
Figure 1 - Schematic diagram of the transfer system in standby mode (a) and cut mode (b).
The core of this multidimensional gas chromatography system is the simple switching device for accurate transfer of an unseparated fraction from the first column to the second column. The interface is thermally stable and is characterized by extremely reduced dead volumes. Figure 1 shows the switching device in two modes: the standby mode and the cut mode. In both configurations, the APC supplies the carrier gas at a constant pressure (P) to the external fused-silica restrictor (termed R1) and to the three-way solenoid valve. The latter is connected to two metallic branches, one with a fused-silica restrictor (termed R2) and one without. The dimensions of R2 (length and internal diameter) generate a pressure drop (ΔP2) that is slightly larger than that due to R1 (ΔP1).
As shown in Figure 1a, when the three-way valve is not activated, the APC pressure is reduced on the side of the first column (P–ΔP1). Meanwhile, the APC pressure is able to reach the second-dimension branch, passing through the solenoid valve, without changes (P). In such a pressure configuration, the analytes from the first column are directed to the first FID. This is called the standby mode. The system is switched to the cut mode (Figure 1b) by simply activating the solenoid valve. The exit pressure of the first column is the same as that described previously (P–ΔP1), while the second column is now P–ΔP2, which is slightly lower than P–ΔP1. Then, the samples eluted from the first column are directed onto the second column.
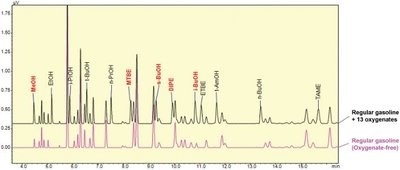
Figure 2 - Chromatogram of the first column. Comparison of the chromatogram of regular gasoline to which oxygenates were added to the chromatogram of oxygenate-free regular gasoline.
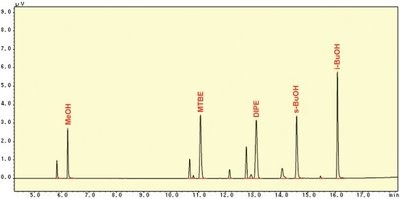
Figure 3 - Chromatogram of the second column.
It must be emphasized that retention time shifting as a result of multiple heart-cuts in a single chromatographic run was negligible. Retention time shifting is a serious issue that can negatively affect analysis and occurs when the exit pressure of the first column in the standby mode differs from that in the cut mode. In this study, because the pressure drop in the primary column remained constant (P–ΔP1) due to design considerations of the multi-Deans switching mechanism, retention time shifting is not a concern.
Figure 2 shows a comparison of the chromatogram of regular gasoline to which oxygenates were spiked to the chromatogram of oxygenate-free regular gasoline. The eight compounds—EtOH, i-PrOH, t-BuOH, n-PrOH, ETBE, t-AmOH, n-BuOH, and TAME—that were completely separated from the complex hydrocarbon matrix by the first column were measured by the first FID. The five compounds—MeOH, MTBE, s-BuOH, DIPE, and i-BuOH—that were insufficiently separated by the first column were transferred to the second column and measured by the second FID. The chromatogram of the second column is shown in Figure 3. It can be observed that five target compounds were determined, completely separated from the complex hydrocarbon matrix.
Table 1 - Repeatability of peak area and retention time
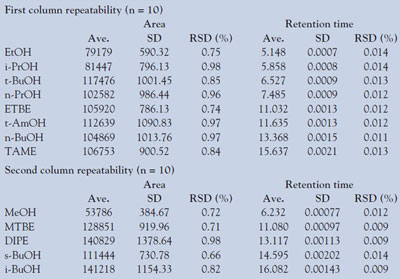
The repeatability of the retention time and the peak area of 13 oxygenates (each 2 m/m%) are listed in Table 1. The values indicate that the accuracy of the MDGC/GCMS-2010 can be equal to that of a monodimensional GC system. A high level of linearity of the 13 compounds (ether: approx. 0.1–20 m/m%, alcohol: approx. 0.1–15 m/m%) was also obtained. The r2 values of all compounds fell in the range of 0.9974–0.9998.
One concern with this method is the analysis run time, which can be as long as 30 min for some gasoline samples. The long run time is mostly attributed to the time needed for the high-boiling compounds to elute from the first column. However, since the system employs an APC unit as the switching device, unnecessary peaks can be backflushed with a high flow rate after the last analyte peak elutes from the first column. After the last peak of the analyte elutes from the column, the switching device goes into the standby mode, the split/splitless inlet pressure automatically decreases from 289.1 kPa to 20 kPa, and the pressure of the APC unit increases from 100 kPa to 300 kPa. Any high-boiling compounds remaining on the first column are backflushed to the split vent. Figure 4 shows the backflush effect on analysis time, which has been reduced to 21 min.
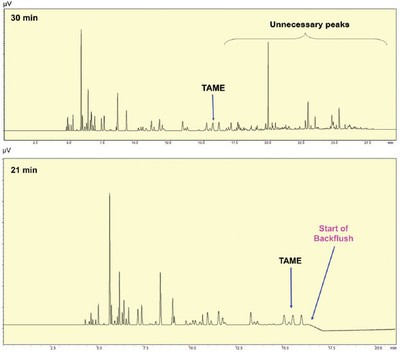
Figure 4 - Contribution of the backflush technique to a reduction in analysis time (chromatogram of the first column after multiple heart-cutting programs).
Conclusion
A multi-Deans switching device was used for heart-cutting unresolved analytes from one column to another so that complete chromatographic separation could be achieved for all target compounds from the hydrocarbon matrix. Excellent repeatability and linearity were also obtained, highlighting the accuracy of the MDGC/GCMS-2010. Finally, a backflush of the first column was employed to reduce the analysis time and improve productivity.
References
- ASTM D4815-99: Standard Test Method for Determination of MTBE, ETBE, TAME, DIPE, tertiary-Amyl Alcohol and C1 to C4 Alcohols in Gasoline by Gas Chromatography.
- Deans, D.R. Chromatographia1968, 1(1– 2), 18–22.
Mr. Hiraoka, Ms. Asakawa, and Mr. Wada are Application Chemists, and Mr. Furukawa and Mr. Terai are Mechanical Engineers, Shimadzu Corp., 380-1, Horiyamashita, Hadano-city, Kanagawa, 259-1304, Japan; tel.: +81 463 88 8660; fax: +81 463 88 8670; e-mail: [email protected].