The use of membrane materials for the extraction of organic compounds has increased during the past few years1–6 for the extraction of analytes in bioanalysis and environmental, food, and industrial analysis. Jönsson and Mathiasson developed the supported liquid membrane (SLM) technique2,6 and described a microporous membrane liquid–liquid extraction (MMLLE).7 SLM extraction is chemically similar to LLE, extracting compounds from an aqueous sample to an organic solvent, and in a second extraction, in a different aqueous phase. The MMLLE technique is a complement to SLM extraction, permitting the extraction to be extended to further classes of compounds. MMLLE is the best method for hydrophobic, uncharged compounds that cannot be easily extracted with SLM.
Petersen-Bjergaard and Rasmussen established a liquid-phase microextraction technique (LPME)8,9 in which the fiber is permeated with an organic phase to create both SLM and MMLLE systems, depending on the nature of the acceptor phase.
In 2001, Hauser and Popp introduced membrane-assisted solvent extraction (MASE),10–15 in which the membrane is composed of dense polypropylene polymer. The principle consists of a liquid–liquid extraction, but at small scale. Membrane extraction used to extract apolar or low-volatility substances is achieved when equilibrium is reached, based on the same principle of LLE. The analytes permeate through the membrane, from the liquid phase to an acceptor phase, according to their partition coefficient in the sample/solvent system as a normal permeation process. The principle of diffusion into the extraction phase is based on the concentration gradient phenomenon and on the Nernst distribution coefficient. The extraction efficiency depends primarily on the distribution coefficient of the analytes between both phases (sample and organic solvent), with the membrane acting as a physical borderline. For low-molecular-weight compounds, the membrane acts like a thin solvent layer and the situation may be compared to a three-liquid-phase system. For high-molecular-weight molecules such as proteins, the membrane acts only as a physical border because the molecules are too large to pass through this solid polymer framework.
A comparison between stir bar sorptive extraction (SBSE) and MASE16 shows that MASE gives better recovery, is simpler and faster, and can also be automated; on the other hand, SBSE has a better detection limit and linearity. Many compounds can be analyzed with this technique, such as organophosphorus pesticides, polychlorinated biphenyls (PCBs), triazine, organochlorine compounds, and volatile compounds,11–15 and extraction recoveries vary from 47 to 100%, depending on the compound.
The aim of this study was to apply MASE for a large range of compounds in order to obtain a simple screening extraction method for complex liquid samples.
Experimental
Chemicals and standards
Methanol and cyclohexane (analytical grade) were purchased from Merck (Darmstadt, Germany); ethyl acetate, dichloromethane, and diisopropylether were from J.T. Baker (Deventer, Holland). Deionized water (18.2 MW) was obtained with a Milli-Q system (Millipore Corp., Bedford, MA). Sodium chloride was purchased from Sigma-Aldrich (Steinheim, Germany) and standards of 99% purity were obtained from Restek (Bellefonte, PA): decane, undecane, dodecane, tridecane, phenol, aniline, 1-octanol, 2-methylphenol, nonanal, N-methylaniline, 2-methylaniline, 2,6-dimethylaniline, naphthalene, 2,6-dichlorophenol, 2,4,6-trichlorophenol, atrazine, and methylstearate.
Membrane-assisted solvent extraction
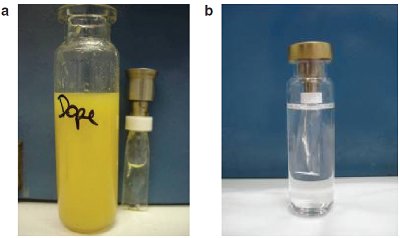
Figure 1 - Experimental setup for MASE: a) orange juice extraction with diisopropylether at 50 °C for 30 min; b) experimental setup. Fifteen milliliters of sample was placed in the headspace vial. The membrane bag was then connected to a PTFE ring and steel funnel introduced into the vial, and 800 μL of organic solvent was added. The vial was closed with a crimp cap.
Membranes (4 cm length, 0.03 mm thickness, and 6 mm i.d.) were from Gerstel (Mühleim, Germany, Figure 1) and were composed of nonporous polypropylene. A 20-mL headspace vial was filled with 15 mL of deionized water spiked with standards or with 15 mL of sample. The membrane bag was attached to a steel funnel with a PTFE ring introduced into the vial, and 800 μL of solvent was added. The vial was then closed with a crimp cap, placed inside the stirring oven for 30 min at 50 °C, and shaken at 750 rpm. After extraction, the vial was removed and the organic solvent was withdrawn and transferred to a 2-mL vial. An aliquot (1 μL) was injected into a GC-MS system.
Apparatus
The separation and identification of organic compounds was performed using an Agilent 6890 GC (Agilent Technologies, Waldbronn, Germany) with an RTX 35 column (30 m × 0.25 mm × 0.25 μm film thickness, Restek) equipped with an MPS 2 multipurpose sampler (Gerstel) and model 5973 mass-selective detector (Agilent Technologies). The split/splitless injector was operated in the splitless mode. The carrier gas was helium at a flow rate of 1 mL/min constant flow. The oven was held at 40 °C for 2 min, and subsequently the temperature was increased to 130 °C at 6 °C/min, and then to 300 °C at 15 °C/min. The detector was operated in the electron impact ionization mode with an ionization voltage of 70 eV in full scan mode from m/z 35 to 350. The ion source temperature of the mass-selective detector and the injector temperature were kept at 250 °C, the quadrupole at 150 °C, and the transfer line at 300 °C.
Results and discussion
In order to optimize membrane extraction, several parameters were considered: mixing speed, extraction time, extraction temperature, salt concentration, methanol addition, solvent, and pH impact. The elastic material of membrane resists most solvents, and is stable at elevated temperature and within a large pH range. In most cases, this extraction shows that 750 rpm agitation gives a better extraction for polar compounds.11 This agitation speed was chosen for all experiments.
Optimization of extraction parameters
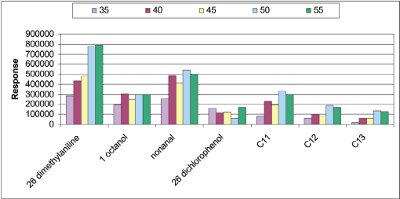
Figure 2 - Temperature influence between 35 and 55 °C of seven standards (extraction with cyclohexane at 750 rpm for 30 min).
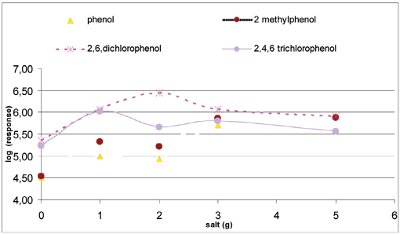
Figure 3 - Salt impact for phenol and its derivatives (extraction with cyclohexane at 750 rpm for 30 min).
- Optimization of extraction time. The extraction time is 30 or 60 min in most cases.12,13 This parameter was then tested from 10 to 60 min at 40 °C with cyclohexane in triplicate. Results show an increase in compound response until 60 min. The longer the extraction time, the more compounds that are extracted. A 30-min extraction time is sufficient, since a longer time does not fit with the authors’ goal of developing a shorter extraction method. This value was retained for the rest of the study.
- Optimization of extraction temperature. The lowest temperature issued by the stirring agitator is 35 °C. Several temperatures were tested: 35, 40, 45, 50, and 55 °C in triplicate with cyclohexane at 750 rpm (Figure 2). The compound responses follow the rate of temperature. High temperature makes mass transfer easier because the diffusion coefficient increases, but at the same time the partition coefficient of the analytes in the membrane decreases. A temperature of 55 °C is not suitable if the vial is not carefully closed, which can induce solvent loss. A temperature of 50 °C was selected, just under the boiling point of the solvent.
- Optimization of salt concentration (salting out). Salt does not pass through the membrane, and in addition has a very significant influence, as shown in Figure 3. The response of most compounds increases when salt addition increases, except for nonpolar compounds such as naphthalene and hydrocarbons, where the responses decrease when salt addition is close to 5 g. Popp et al. showed that salting out is not necessary for phenanthrene and hexachlorocyclohexane (HCH).11 For a log Kow between 1 and 2, salt addition increases compound response, but with a log Kow above 5.25, it is harmful. An addition of 3 g of salt has been chosen for the following experiments to extract polar compounds.
- Methanol impact. Methanol addition is often necessary to prevent the adsorption of compounds on the glass wall of the extraction vial, which is why the addition of methanol has also been tested. The curves of N-methylaniline, aniline, 2,6-dimethylaniline, and 2-methylaniline level out at 10%, whereas the curves of phenol and atrazine rise and decrease after 5%. In this case, and for the investigated compounds, the results of methanol addition show no significant variation.
- Solvent choice. In most studies, the solvents used with this technique were hexane, cyclohexane, heptane, diisopropylether, chloroform, and ethyl acetate.11–15 Methanol,12 chloroform, and diethyl ether are not suitable because they diffuse through the membrane in the aqueous phase. Three solvents were tested: cyclohexane, ethyl acetate, and diisopropylether. Solvent efficiency varied among the compounds. Hydrocarbons such as decane or dodecane were extracted with ethyl acetate; for aniline and its derivatives, the best choice was diisopropylether.
- pH impact. Because sample pH affects the dissociation equilibrium of compounds in aqueous sample, the effect of pH variation has been examined. Consequently, sample pH was also tested, and varied over the range from 2 to 10. Results showed that most compounds led to a better extraction at pH 2, except aniline and its derivatives. At low pH values, acidic compounds are neutralized forms, which makes it easier to extract them. The responses of aniline and its derivatives increase greatly with the pH because these compounds are more basic. Consequently, use of a single method for satisfactory extraction of all compounds is not possible.
Real sample analysis
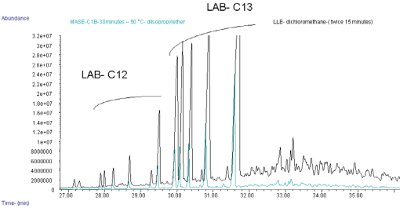
Figure 4 - Chromatogram of industrial wastewater analysis (extraction time: 30 min, extraction temperature: 50 °C, solvent: diisopropylether) obtained in scan mode (m/z 35–350).
An example of wastewater obtained from an industrial water treatment plant was extracted for 30 min with diisopropylether and emphasized several compounds such as alkyl benzene linear LAB, which stems from alkyl benzene sulfonate (LAS) (Figure 4). A comparison between liquid–liquid extraction with dichloromethane for 30 min and MASE showed the same compounds. MASE allows simple and fast extractions.
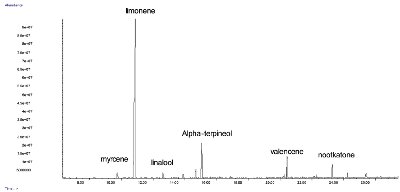
Figure 5 - Chromatogram of orange juice membrane extraction (extraction time: 30 min, extraction temperature: 50 °C, solvent: diisopropylether).
Orange juice, purchased at a local market, was extracted with 800 μL of diisopropylether for 30 min at 50 °C. Again, for this complex matrix, several compounds were extracted (Figure 1 and Figure 5) and there was no need for cleanup.
Conclusion
The sample preparation and free solvent methods continue to be studied. MASE is easy to apply and fast to perform. It is an attractive alternative for complex samples, since there is no emulsion, cleanup is avoided, and membranes can be reused after cleaning. The best determined conditions for this study are 50 °C for 30 min with diisopropylether. Solvent choice is the most significant factor in this technique and should be adapted according to the compound family. Extraction recovery can increase with salt addition: 3 g NaCl proved to be the best choice for all polar compounds. A basic extraction is necessary for aniline and its derivatives, and methanol addition is useless. This analytical procedure was successfully applied to the characterization of complex matrices such as industrial wastewater. The extraction is a useful approach to identifying organic compounds in complex matrices.
References
- Pawliszyn, J. Comprehensive Analytical Chemistry; Jönsson, J.A., Ed.; Elsevier Science B.V.: Amsterdam, The Netherlands, 2002; 37; pp 389–477.
- Jönsson, J.A.; Mathiasson, L. Liquid membrane extraction in analytical sample preparation. II. Applications. Trends Anal. Chem. 1999, 18(5), 325–30.
- Urtiaga, A.M.; Ortiz, M.A. Supported liquid membranes for the separation-concentration of phenol. 2. Mass-transfer evaluation according to fundamental equations. Ind. Eng. Chem. Res.1992, 31, 1745–8.
- Chimuka, L. Speciation studies of metal ions in environmental and biological media using supported liquid membrane extraction. LC-GC Eur. Jul 2004, 396–5.
- Khrolenko, M.; Wieczorek, P. Determination of glyphosate and its metabolite aminomethylphosphonic acid in fruit juices using supported-liquid membrane preconcentration method with high-performance liquid chromatography and UV detection after derivatization with p-toluenesulphonyl chloride. J. Chromatogr. A2005, 1093, 111–6.
- Jönsson, J.A.; Mathiasson, L. Membrane extraction in analytical chemistry. J. Sep. Sci. 2001, 24, 495–12.
- Jönsson, J.A.; Mathiasson, L. Liquid membrane extraction in analytical sample preparation. I. Principles. Trends Anal. Chem. 1999, 18(5), 318–7.
- Petersen-Bjergaard, S.; Rasmussen, K.E. Liquid-liquid-liquid microextraction for sample preparation of biological fluids prior to capillary electrophoresis. Anal. Chem. 1999, 71, 2650–6.
- Pedersen-Bjergaard, S.; Rasmussen, K.E. Liquid phase microextraction utilising plant oil as intermediate extraction medium—towards elimination of synthetic organic solvents in sample preparation. J. Sep. Sci. 2004, 27, 1511–6.
- Hauser, B.; Popp, P. Membrane-assisted solvent extraction of organochlorine compounds in combination with large-volume injection/gas chromatography-electron capture detection. J. Sep. Sci. 2001, 24, 551–9.
- Hauser, B.; Popp, P.; Kleine-Benne, E. Membrane-assisted solvent extraction of triazines and other semi-volatile contaminants directly coupled to large-volume injection-gas chromatography-mass spectrometric detection. J. Chromatogr. A2002, 963, 27–9.
- Schellin, M.; Popp, P. Membrane-assisted solvent extraction of seven phenols combined with large volume injection-gas chromatography-mass spectrometric detection. J. Chromatogr. A2005, 1072, 37–6.
- Schellin, M.; Popp, P. Membrane-assisted solvent extraction of polychlorinated biphenyls in river water and other matrices combined with large-volume injection-gas chromatography-mass spectrometric detection. J. Chromatogr. A2003, 1020, 153–7.
- Schellin, M.; Hauser, B.; Popp, P. Determination of organophosphorus pesticides using membrane-assisted solvent extraction combined with large volume injection-gas chromatography-mass spectrometric detection. J. Chromatogr. A2004, 1040, 251–7.
- Hauser, B.; Schellin, M.; Popp, P. Membrane-assisted solvent extraction of triazines, organochlorine, and organophosphorus compounds in complex samples combined with large-volume injection-gas chromatography/mass spectrometric detection. Anal. Chem.2004, 76, 6029–11.
- Gomes Zuin, V.; Schellin, M. Comparison of stir bar sorptive extraction and membrane-assisted solvent extraction as enrichment techniques for the determination of pesticides and benzo[a]pyrene in Brazilian sugarcane juice. J. Chromatogr. A2006, 1114, 180–7.
Mrs. Vincelet is Researcher, and Mr. Benanou is Manager of the Analytical Research Dept., Veolia Environnement, Centre de Recherche sur l’eau, Chemin de la Digue, 78600 Maisons-Laffitte, France; tel.: +33 1 34 93 81 19; e-mail: [email protected]. Dr. Roussel is Associate Professor, University of Paris XI, Paris, France.