Ion exchange is particularly well suited to the separation
of biological molecules; however, limitations
of HPLC packing materials have restricted its use.
Silica-based ion-exchange materials are pH limited
and suffer from nonspecific interactions, although
they can have high efficiency. Porous, polymer resin-based
materials may be used over a wide range of pH
and have a high capacity (dynamic binding capacity
~45 mg/mL); however, they exhibit lower efficiency
and have pressure limitations. Nonporous resins
with fast mass transfer characteristics are efficient
but have extremely low capacity (<5 mg/mL). The
desire to analyze large numbers of charged components
in proteomic samples has driven the search for
improved ion-exchange columns.
Experimental
A nonporous, polymer resin particle was modified to
address the needs of ion-exchange separation. First,
a 200-Å-thick hydrophilic layer was chemically
bonded to the polystyrene/divinylbenzene (PS/DVB)
surface to virtually eliminate nonspecific binding
with biological molecules. Then, a densely packed,
uniform ion-exchange layer was chemically synthesized
to the hydrophilic layer. Multiple ion-exchange
functional groups were attached to one anchoring
point, resulting in a breakthrough in capacity. The
four varieties (SAX, WAX, SCX, and WCX) have
dynamic binding capacities ranging from ~20 mg/mL
to over 50 mg/mL—for example, 20, 38, 53, and 65
mg/mL for Proteomix SCX-NP resins (Sepax Technologies,
Newark, DE) with particle sizes of 10, 5, 3,
and 1.7 μm, respectively.
Separation efficiency can be described in terms of
plate height, HT in Eq. (1).1 Efficiency is defined as the
standard deviation (σ2) divided by the bed or column
length (L), and it is the sum of HEddy, HMT, HSMT, HLD,
HSNS, and HSSP, which are plate heights contributed
by eddy diffusion, mobile phase mass transfer, stagnant
mobile phase mass transfer, lateral diffusion, stationary
phase nonspecific interaction, and stationary
phase specific interaction, respectively.

Eliminating the pores minimizes the contribution
from stagnant mobile phase mass transfer. The
hydrophilic layer makes the nonspecific interaction
factor negligible.
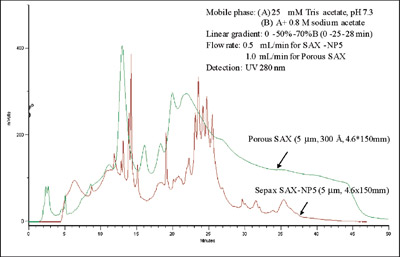
Figure 1 - Separation of horse serum (20 μL, 2× diluted) on 300-Å porous SAX
column compared to nonporous Proteomix SAX.
Figure 1 compares the separation of horse serum
on a traditional 300-Å porous ion-exchange column
to that achieved on a nonporous Proteomix
SAX. Both are 5-μm, 4.6 × 150 mm columns.
Capacities are equivalent, but efficiency and resolution
are significantly improved on the nonporous
resin.
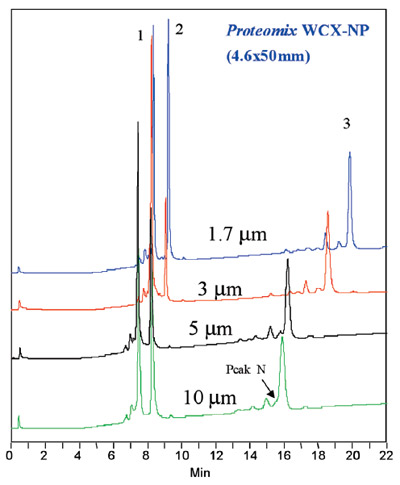
Figure 2 - Separation of a protein mixture using four
Proteomix WCX-NP columns with particle size ranging from
1.7 μm to 10 μm. Separation conditions—Mobile phase A:
20 mM phosphate-buffered saline (PBS), pH 6.5. Mobile
phase B: A + 1.0 M NaCl. Gradient: 0–100% B in 20 min.
Flow rate: 1.0 mL/min. Pressure: 10 μm, 540 psi; 5 μm,
890 psi; 3 μm, 1600 psi; 1.7 μm, 3100 psi. Detection: UV
at 280 nm. Proteins (1.0 mg/mL each): 1) ribonuclease A,
2) Cytochrome C, 3) lysozyme.
Particle size also plays an important role in efficiency
and resolution. Decreasing particle size
increases efficiency but dramatically increases
backpressure. Commercialization of ultrahigh-pressure LC instrumentation enables the use of
very small particles.2 Traditional resin-based ion-exchange
materials have been unable to withstand
the additional backpressure. The upper
pressure limit for Proteomix nonporous packings
is 10,000 psi, which enables their use in UPLC™
(Ultra Performance LC™) systems (Waters, Milford,
MA) and other high-pressure systems. Figure
2 shows a separation on four different particle
sizes of Proteomix WCX-NP from 10 μm to 1.7
μm. Note the resolution of the small peak adjacent
to lysozyme.
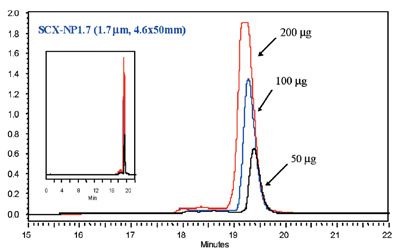
Figure 3 - Loading capacity test elution of Cytochrome C (20 mg/mL).
Separation conditions—Mobile phase A: 20 mM PBS. Mobile phase B: A + 1.0
M NaCl. Gradient: 0–70% B (21 min). Flow rate: 0.35 mL/min, 2800 psi.
Detection: UV at 280 nm.
A loading experiment using Cytochrome C, shown
in Figure 3, demonstrates a high capacity
while maintaining performance for the
1.7-μm nonporous material.
Conclusion
The use of nonporous resins improves
mass transfer and allows smaller particle
sizes to dramatically improve
efficiency in any pH range. Chemical
modification to eliminate nonspecific
binding and optimize ion-exchange
capacity overcomes the limitations
of classical nonporous resins. Ion-exchange
chromatography using nonporous,
specially modified resin-based
packings should become
a widely used tool for the
analysis of large numbers of proteins, low-abundance
protein detection,
and monitoring of
protein post-translational modification,
as well as the analysis of other
biological molecules. They should
also find use in pharmaceutical
applications in which small ionic
solutes can be difficult to retain by
reversed phase.
References
- Giddings, J.C. United Separation Science;
John Wiley & Sons, Inc.: New York,
1991; Chap 12; pp 269–89.
- Mazzeo, J.R.; Neue, U.D.; Kile, M.; Plumb, R.S.
Advancing LC performance with smaller particles and
higher pressure. Anal. Chem. A-pages 2005, 77(23),
460A–7A.
Dr. Huang is CTO, and Ms. McKay is Director of Marketing,
Sepax Technologies, Delaware Technology Park, 5-100
Innovation Way, Newark, DE 19711, U.S.A.; tel.: 302-366-1101; fax: 302-366-1151; e-mail: [email protected].