Introduction of gas chromatography samples typically occurs through a heated inlet containing a glass liner. The role of the inlet liner is to reproducibly transfer the sample, in a gaseous phase, to a column where the chromatographic separation occurs. Use of a proper inlet liner is critical to ensuring the best possible chromatography. Inlet liners are available in a wide variety of configurations, which often makes selection difficult. The best inlet liner for an analysis depends on a number of factors, including type of GC inlet, nature of the sample and chemical properties of the analytes.
Liner geometry
The following sections provide recommendations for liners based on the type of analysis. No single liner is perfect, and sometimes compromises must be made. Figure 1 shows commonly used liners by analysis type.
Figure 1 – Liner configurations by analysis/injection type.Gas analysis
Some GC analyses feature a sample that is already in the gaseous form upon introduction into the inlet. These analyses include direct gas injections, purge-and-trap, headspace, air sampling and thermal desorption. Since the inlet does not need to vaporize the sample in this case, the liner’s main role is the quick, efficient transfer of the sample to the column. A straight, narrow-internal-diameter (1-mm) liner is often the best choice for this purpose. The small internal diameter of the liner results in higher linear velocities of the carrier gas as it passes through the liner, rapidly transferring the analytes to the head of the column. This prevents excessive diffusion, which can lead to broadened peaks.
Splitless analysis of liquid extracts
Unlike analyzing gas samples, where the liner’s only role is to transfer the sample to the column, extracts require the inlet to sufficiently and reproducibly vaporize liquids prior to column loading. During a splitless injection, the GC’s split vent is closed, directing all flow to the column. This results in longer residence times and slower flow rates in the liner.
Liners used for splitless analysis include single taper liners with and without wool, straight liners with and without wool and other less common configurations, such as a double taper Cyclo liner (Restek Corp., Bellefonte, Penn.). Figure 2 shows a comparison using hydrocarbons ranging from C8 to C40 to demonstrate performance and reproducibility of these liners over a wide compound volatility range. As shown, a single taper liner with wool is one of the best liners for splitless analyses. The wool enhances vaporization of heavier analytes (due to its large surface area and high heat capacity) and aids in the mixing of the sample with the carrier gas to lead to better reproducibility. Wool also acts as a type of filter to capture nonvolatile matrix components and prevent them from entering the column. In addition, wool helps to “catch” the sample and prevent less-volatile analytes from missing the column and hitting the bottom inlet seal, where activity can occur or the sample can condense out and eventually be swept out of the split vent once the splitless hold time ends. The bottom taper directs the sample to the column and decreases the chance of contact with the hot inlet seal.
Figure 2 – Comparison of liner configurations over a wide-molecular-weight range of hydrocarbons for splitless injections. The single taper liner with wool achieves the best responses for heavier compounds and has excellent reproducibility with <2% RSD for all compounds over seven injections.Split analysis of liquid extracts
During a split injection, flow is directed both to the column and out of the split vent at a predetermined ratio. This is ideal for concentrated or “dirty” samples, where the analyst only wants to sample a portion of the injected sample; it is essentially a dilution occurring in the inlet. Because the flow is split at the bottom of the inlet, the actual carrier gas flow going through the liner is much higher when using split injections (the total of the split vent flow and the column flow). This produces faster, more efficient loading of the sample onto the column, resulting in narrow, well-defined peaks. Adverse interactions within the liner are greatly reduced since the time the analyte spends in the liner is short compared to splitless injections.
Though setting up a split method is generally easier than splitless, proper liner choice is essential to achieving reproducible and complete, nondiscriminatory vaporization. Split liners should have an open bottom, rather than a taper, as this allows for accurate splitting. Wool or some kind of additional obstruction is critical with split liners to provide mixing of the sample with the carrier gas and additional surface area to enhance vaporization. Without some kind of obstruction, upon injection, the high flows in the liner will direct much of the sample to the bottom of the inlet before complete vaporization, resulting in poor reproducibility. Figure 3 shows a performance comparison for several commonly used split liners. A straight liner without wool, though not recommended, is included to demonstrate the importance of having wool or another obstruction.
Figure 3 – Comparison of liner configurations over a wide-molecular-weight range of hydrocarbons, using a 50:1 split injection. The Precision liner with wool has the overall highest responses and lowest %RSDs.Useful in split injections, Precision-style liners (Restek) feature a plug of glass wool held in place by baffles and an open-bottom design. Sample is injected into the wool, ensuring thorough mixing and vaporization. In addition, the needle is wiped of any residual droplets as it is removed from the wool, leading to increased reproducibility. A straight liner with wool can provide similar performance, but loses the benefit of needle wiping, and there is an increased chance that the wool can move around with pressure changes, reducing precision. Other liners used for split injections feature glass obstructions, such as a cup or corkscrew (Cyclo). These formations serve a similar purpose to the wool as regards mixing and evaporation, but the liners are generally costlier since they are more difficult to manufacture. Some analysts prefer these liners for active compound analysis since they do not have wool, but activity from woolpacked liners is generally less of a problem when using split injections because of the very short liner residence times of the analytes.
Programmed temperature vaporization
A programmed temperature vaporization (PTV) inlet has the ability to change temperatures upon injection. This is often used for large-volume injections with solvent venting, where the inlet can initially be held at a low temperature while the sample is slowly injected, allowing the solvent to “vent” off while analytes are retained. Once most of the solvent has been vented, the inlet can be rapidly heated to vaporize and transfer analytes to the column. PTV inlet liners commonly feature baffles or similar obstructions designed to catch the sample upon injection and prevent it from being immediately vented. The solvent can then be evaporated from the baffle surface prior to closing the vent and the temperature ramped to vaporize analytes of interest. Baffles provide increased surface area for enhanced vaporization and can create turbulence to aid with sample mixing.
Deactivation
Liners are most often made of borosilicate or quartz glass and can contain active sites such as silanols and metals, which can lead to chemical reactions and adsorption of analytes within the liner. To prevent these adverse reactions, liners must be deactivated. This is essentially a silanization of the surface.
Several methods are used to deactivate liners. While some researchers may prefer to use their own technique, a few GC consumables’ manufacturers offer robust liner deactivations that have very inert surfaces, resulting in improved responses for active compounds, even at trace levels. The deactivation techniques used by these companies are often more advanced than can be achieved with standard laboratory equipment.
A comprehensive deactivation is especially critical to the use of glass wool, as the large surface area of wool can have many active sites. Some analysts avoid using wool because it is difficult to deactivate thoroughly, leading to significant activity. Several manufacturers offer liners prepacked with wool, some of which feature excellent deactivations that work well, even for active compounds at trace levels. Both borosilicate and quartz wool are used; however, quartz has fewer impurities and as a result has superior performance. It is not recommended that analysts pack their own wool, as fibers can break, exposing active sites. Table 1 demonstrates the differences in sensitive compound response of a comprehensive deactivation with quartz wool versus a subpar deactivation with borosilicate wool in splitless mode. The drastic differences in responses demonstrate the effect of liner deactivation and wool type on sensitive analyte recovery.
Table 1 – Comparison of splitless low-level pesticide response factors on two liner deactivations, one containing quartz wool and the other borosilicate wool, both in single taper format
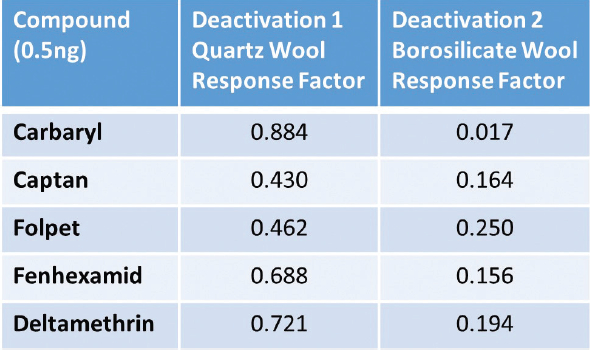
Volume
Many liner configurations are offered with multiple internal diameter options, or volumes. In general, a smaller internal diameter will transfer analytes to the column in a tighter band and lead to narrower peaks; however, when a solvent is injected into a hot inlet, a rapid expansion occurs as it is vaporized. At low inlet pressures, this can cause the sample to expand beyond the liner into carrier gas lines and other open spaces, potentially leading to activity, contamination, ghost peaks and poor reproducibility. Higher inlet flows, like those used in split analyses, reduce these problems.
All solvents have different expansion coefficients and the analyst must determine an appropriate liner volume based on their solvent, injection volume, inlet temperature and pressure. There are free calculators available online for calculating the expansion volume of various solvents at a given temperature, injection volume and column head pressure. Liner volume can be estimated using the formula for the volume of a cylinder (V = πr2h) or by contacting the manufacturer.
Conclusion
The number of inlet liners available for gas chromatography can be overwhelming. Liner choice can be greatly simplified by considering sample type, injection mode and the nature of the analytes, such as activity and volatility. Use of an appropriate liner for a particular analysis can help improve peak shapes, detection limits and reproducibility. In summary, some of the best liner choices include a narrow internal diameter straight liner for gas analysis, a single taper liner with wool for splitless analysis, a Precision-style liner for split analysis and a baffled liner for PTV analysis.
Linx Waclaski is GC accessories chemist, Restek Corp., 110 Benner Cir., Bellefonte, Penn. 16823, U.S.A.; tel.: 814-353-1300; e-mail: [email protected]; www.restek.com