Chiral molecules continue to drive
growth across a number of industries as
new chemistries, active ingredients, and
products are developed and commercialized.
These industries include pharmaceuticals,
fine chemicals and intermediates,
agrichemicals, and flavors and
fragrances, as well as emerging nutraceutical
and cosmeceutical areas. Due to significant
benefits provided by single-enantiomer
therapeutics and by FDA
requirements, the pharmaceutical industry
in particular has been actively driving
chiral chemistry and technology.
But for all the advantages of chiral compounds,
isolating and purifying them
has remained a difficult, inefficient, and
costly proposition. The technology discussed
here approaches chiral separations
in a radically different way. A new
class of “smart” nano structured materials
have been developed that are highly
effective at separating a broad spectrum
of chiral chemical compounds. This
technology, called EnantioSelective
Polymer™ (ESP™, Evolved Nanomaterial Sciences [ENS], Cambridge,
MA), comprises a suite of novel chiral-selective
materials that can be used in a
variety of industry formats to deliver
chiral separation solutions.
Chiral drugs are one of the fastest-growing
categories of pharmaceuticals. Each
molecule of a chiral drug has a mirror
image. These mirror image molecules, or
enantiomers, are identical in terms of their
chemical structure and physical properties.
While enantiomers can be very hard
to distinguish using chemistry, they can
have very different biological effects, since
the physiological environment is also predominantly
chiral. A chiral drug containing
a mixture of enantiomers may contain
two or more distinct biological ingredients,
each with different biological activities.
This can cause problems with dosing,
lower effectiveness for the chiral drug,
unpredictable pharmacokinetics, or harmful
side effects. For some chiral drugs to be
effective, the mirror image isomer must be
eliminated. Since the enantiomers are
chemically identical, and differ only in
their three-dimensional geometry, selectively
eliminating the undesirable enantiomers
can be very challenging.
Several different conventional avenues
exist to separate enantiomers from a chiral
mixture. Chiral crystallization methods,
including diastereomeric crystallization,
have been around for decades, but method
development is tedious and success is
often “hit or miss.” On the other hand,
chiral chromatography has a higher rate of
success and is growing in popularity.
The difficulties that exist in chiral separations
have spurred a continued interest in
chiral-selective materials that can be
used as stationary phases. Most of the
current chiral materials select for a particular
enantiomer by weakly binding it to
another chiral molecule that is immobilized
on a support within a column. The
chiral molecule, or selector, plus the support,
make up the chromatographic stationary
phase. Different enantiomers are
retained by the stationary phase with different
kinetic time scales, resulting in a
separation. In most cases, the stationary
phase incorporates a large, complex
molecule that can stick to the analyte
enantiomers at multiple points, leading
to chiral selectivity. In other cases, a
folded protein is stuck to the support, and
analytes are captured in the chiral-shaped
pockets of the protein and are
held through hydrogen bonds and other
complexation interactions. Still other
stationary phases rely on beads comprised
entirely of polymers of chiral-selective
molecules, eliminating the need for a
support. All of these approaches rely on a
set of chemical interactions and binding
events to detect the difference between
chiral enantiomers.
EnantioSelective Polymer uses nanotechnology
to directly sense the
three-dimensional chiral geometry of
a molecule by using physical interactions
rather than chemical binding.
The phenomenon is somewhat analogous
to the shape effects observed in
chiral-imprinted polymers, where chiral
molecules in a polymer are dissolved
away, leaving chirally shaped cavities that can select for enantiomers.
Polymer imprinting does not
work well in the absence of binding
interactions, because the shape of the
polymer molecules includes nonchiral
voids or free volume that “washes out”
the imprinted shape recognition. By
using nanoscale engineering techniques,
the company has created a
nanostructured polymer that realizes
the true capability of a physical, “chirally
imprinted” approach. ESP incorporates
a very large number of interconnected
chiral voids. The walls of
the voids are very dense, preventing
the polymer free volume from degrading
the chiral selectivity of the chiral
voids. Interconnecting the voids
makes much more of the material
accessible to analyte molecules. ESP
medium has an ultrahigh interior surface
area comprising minute channels
larger than the size of a large
molecule. The channels are tightly
packed with chiral molecular chains
that protrude like dense brushes. Diffusing
molecules are forced to interact
with these brushes because the interstices
are too small to allow any other
pathway through the material. The
chiral brush and chiral shapes of the
channels select for the enantiomers.
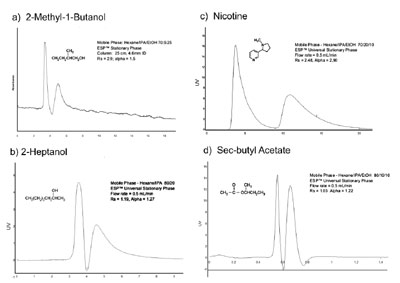
Figure 1 - Using ESP technology, the same stationary phase and identical column can separate
enantiomers of two alcohols (a and b), an alkaloid (c) that causes adsorption problems in conventional
HPLC media, and a small acetate (d). Other compound classes such as fatty chain molecules,
acids, and amines have also been separated using the same stationary phase. Small molecules such as
the acetates and alcohols shown (a, b, and d) often present challenges for chromatographic separation.
In contrast to chiral materials that select
for specific molecules or classes of
molecules, ESP has a broadly selective
spectrum. For example, the identical
ESP-packed HPLC column can separate
chiral alcohols, free amines, chiral acids,
terpenes, alkaloids, and amino acid
derivatives, as illustrated in Figure 1. A
number of compounds within these
classes, as well as entire classes such as
small alcohols and hydrocarbons, have
proven very difficult to separate employing
conventional HPLC column technology.
These compounds and compound
classes can be successfully
resolved with ESP, and are represented
in Figure 1. Several HPLC traces, all
using the identical column without
repacking or a change of stationary
phase, are shown in the figure. It is
important to note that the solvents used
for ESP HPLC separations are mild and
environmentally friendly: typically,
ethanol, isopropanol, and hexane.
In addition to chromatographic separations,
the polymer can be used as
a batch sorbent or as a chiral molecular
sieve. This characteristic is
unusual and extremely powerful;
ESP is indeed a “smart” material and
allows for different separation techniques
to be utilized in order to
obtain a pure enantiomer. The same
material used in a columnlike device
can also be used as a sorbent. In a
number of cases, the polymer has
been used to achieve high enantiomeric
excess (EE) in a single
batch sorbent step using a breathtakingly
simple approach. The enantiomeric
mixture is poured into a
container as a neat liquid or greater
than 5 wt % solution. ESP with a
150–250 μm particle size is added to
the jar. The ratio of ESP used to
undiluted enantiomers ranges from
1:1 to 5:1 by weight. Once the powdered
ESP has been added to the
container holding the enantiomers
(as neat liquid or a reasonably concentrated
solution), the container is
sealed and agitated for roughly 3–5
min. The ESP is filtered off, leaving
predominantly one enantiomer
behind. To avoid confusion with the
theoretical plates in a chromatography
column, the company refers to
this procedure as a single sorbent
stage. In many cases, greater than
95% EE can be attained in one to
five sorbent stages.
This type of simple, highly scaleable
separation has been demonstrated
for phellandrene, nicotine, several
amino acids and their derivatives,
lactic and malic acid, and a number
of terpenes. The terpenes make a
very useful demonstration because
chiral compounds such as limonene
are inexpensive, not dangerous, and
have commercially available enantiomers
for comparison. In a typical
demonstration, observers are invited
to smell the racemate, which is subsequently
added to two vials of ESP.
One ESP has been designed to
exclude the (+) enantiomers; the
other excludes the (–) enantiomers.
Once the racemate has been added
to the two vials containing the two
ESP types, the vial contents are
mixed by tapping. Observers are
then invited to smell the individual
enantiomers. Typically, a high EE,
greater than 70%, is required to
begin to distinguish enantiomers of
a fragrance. A distinct difference
requires a higher EE. In order to perform
a successful smell test demonstration,
the liquids must also be
added neat, since solvents would
interfere with the enantiomers’ individual
fragrances. A high loading of
neat racemate is also required in
order to wet the ESP enough to
allow mixing. This simple demonstration
establishes the enantio
selective power, speed, and capacity
of this technology.
The manufacturer is applying this
technology to deliver novel tools
for the analytical, synthetic, or process
chemist working with chiral
compounds in a variety of applications,
across many industries. The
ESP suite of technologies provides
breakthrough performance addressing
the many challenges posed by
chiral compounds.
Dr. Valluzzi is Chief Scientific Officer, and Dr.
Chaloner-Gill is V.P. Chromatography,
Evolved Nanomaterial Sciences (ENS),
675 Massachusetts Ave., 14th floor, Cambridge,
MA 02139-3309, U.S.A.; tel.: 617-441-5107; fax: 617-902-2700; e-mail: [email protected].