Abstract
There is growing interest in the use of monodisperse particle-size distribution or PSD (here defined as having 10% RSD or less) to prepare modern HPLC columns because good correlation has been observed between narrow PSD and higher efficiency, longer life and lower pressure drop. HPLC evolution has included synthesis of spherical silica particles with uniform diameters. Monodisperse, nonporous silica (NPS) has been commercially available for 15 years or more; however, columns with NPS particles have not found acceptance in HPLC methods because surface area is too low to retain and resolve most samples. Fused-Core spherical silica with monodisperse PSD was introduced by Advanced Materials Technology (Wilmington, Del.) in 2006. Featuring a uniform, impervious core surrounded by multiple layers of colloidal-size microspheres that provide increased sample capacity, Fused-Core silica has become widely adopted in HPLC methods to improve separation speed.
Another recent example of this trend toward narrower PSD was the introduction of Titan monodisperse, totally porous, spherical silica in 2012 by the Supelco Division of Sigma-Aldrich (St. Louis, Mo.). This article describes the performance of monodisperse silica particles for HPLC and UHPLC and offers thoughts about future direction in HPLC technology.
Introduction to Modern HPLC Particle Design
Use of smaller particles to increase HPLC column efficiency and achieve faster separation was predicted in 1969 by Knox.1 However, it was slow to develop2 because small particle columns and instruments designed to use them were not widely available. The modern era of small-particle HPLC or UHPLC with emphasis on faster separation at high flow velocity and pressure began with the successful introduction of 1.8-µm Zorbax Rx particle columns from Agilent Technologies (Santa Clara, Calif.) in the early 2000s and continued in 2005 when Schwartz3 described the commercial, turnkey Acquity system (Waters Corp., Milford, Mass.); it included a low-dispersion, higher-pressure instrument (ca. 1000 bar) designed to use small i.d. columns filled with 1.7-µm, totally porous silica particles.
An alternate approach to higher HPLC column efficiency and separation speed was described in 2007 by Kirkland,4 who introduced a 2.7-µm superficially porous silica particle under the trade name Fused-Core (Advanced Materials Technology, Wilmington, Del.) silica. The larger particles, with an optimized porous layer and extremely narrow PSD, generated efficiency comparable to sub-2-µm porous particles at lower operating pressure. Fused-Core columns with larger i.d.s could transform traditional instruments having 400‒600 bar pressure limits into much higher-performance systems. Only minor modification to sample inlet and outlet tubing of traditional instruments was required to reduce extra-column bandspreading. An excellent paper by Chester5 was published on this topic in 2009. Modern columns using new solid-core and porous silica particles with classic stationary phases are being rapidly incorporated into new HPLC and UHPLC methods that provide much higher sample throughput.6
Figure 1 ‒ Plots (h-u
) demonstrating a performance advantage for the monodisperse 2.7-µm Fused-Core C8 silica column over a broader PSD 2.5-µm porous column. Sample: cephalosporin antibiotic, Vantin (MW 557.6); columns: 50 × 4.6 mm; mobile phase: 35% acetonitrile/65% 20 mM
sodium phosphate, pH 3.5; temperature: 24 °C. Reproduced with permission from Ref. 4.Figures from Kirkland’s landmark paper4 emphasized two performance advantages for modern core-type particles, including higher efficiency at any velocity and the ability to better maintain efficiency as velocity increases. Figure 1 compares van Deemter plots (see Appendix) for core-type and traditional porous silica of the same particle size for a medium-sized, polar drug, while Figure 2 shows Fused-Core particles breaking the reduced plate height barrier of 2 for the first time (hmin = 1.5) with small molecules. The practical significance of a lower reduced plate height is that higher efficiency can be achieved with larger particle columns that operate at lower pressure. Note in Figure 2 that naphthalene and lorazepam, with different physical and chemical properties, achieve maximum efficiency at different flow velocities and therefore could not be optimized together in the same mixture. During method development, all critical pairs should be tested for efficiency because some pairs may lose resolution faster than others and limit separation speed. In addition to H or N, plotting selectivity (α) and resolution (Rs) versus flow velocity can be a valuable tool for identifying solutes that limit separation speed. Operation at extreme flows and pressures can damage column performance; however, if columns are not rugged at the desired flow velocity, they should not be selected for high-speed methods. Critical pairs with similar structures are less likely to be a problem than solutes having structural differences. Although solute-dependent kinetic behavior was shown by Kirkland for Fused-Core columns, it has been observed for all HPLC and UHPLC silica-based columns, especially in reversed-phase mode. Giddings has described this behavior in terms of adsorption-desorption kinetics and diffusion-controlled kinetics.6
Figure 2 ‒ Plots (h-u
) for two different solutes on a 2.7-µm Fused-Core C8 silica, 50 × 4.6 mm column showing solute-dependent velocity optima. Samples: naphthalene (MW 128.2); mobile phase: 60% acetonitrile/40% water; lorazepam (MW 321.2); mobile phase: 30% acetonitrile/70% 20 mM
sodium phosphate, pH 3.5; temperature: 24 °C. Reproduced with permission from Ref. 4.
Results and discussion
Potential advantages for monodisperse, porous silica
Early theory by Giddings strongly supported the idea that columns with uniform particles should be more efficient and consistent.6 In 1999 and 2002, Knox7,8 revisited the van Deemter relationship and proposed that significant performance gains could still be made by improving HPLC column-bed uniformity. This suggested that some of the performance advantages for core-type particles might be explained by its unique, narrow PSD of only 10% RSD; porous particles typically vary in PSD between 20 and 30% RSD or more. Earlier experiments9 with porous particles had not conclusively established performance advantages for narrow PSD silica; however, the success of core-type particles renewed interest in narrow PSD10 as a possible means to improve performance of porous silica columns.
Figure 3 ‒ Kinetic plots (h-u) for 1.9-µm Titan porous and 2.7-µm Fused-Core C18 columns demonstrating comparable, high performance for the two monodisperse particles; a broader-distribution 1.7-µm C18 column with lower performance is shown for comparison. Sample: uracil, diazepam, toluene, naphthalene, biphenyl; columns: 50 × 3.0 mm; mobile phase: 60% acetonitrile/40% water; temp: 35 °C, flow rate: variable; detection: 254 nm.The first commercial porous silica to feature a narrow PSD that was equal to Fused-Core silica was described in 2012 for 1.9-µm, Titan C18 particles.11 Figure 3 demonstrates reduced plate heights below 2 (h = 1.7) at the optimum velocity for monodisperse, totally-porous silica C18 columns. The van Deemter plots for small molecules are shown to nearly overlap for the two columns with monodisperse particles, indicating that monodispersity may be an important performance factor; a column with broader distribution porous particles is shown for comparison. Figure 4 compares particle distributions for monodisperse Titan and Fused-Core silica to commercial porous silicas; particle samples were removed from new commercial columns for testing.
Figure 4 ‒ Particle size distribution (A) for different silicas with an SEM photograph (B) showing monodisperse Titan silica.Titan column performance
Titan porous silica particles employ a novel process that does not require a secondary sizing operation, allowing the entire lot of silica or modified silica to be utilized in column preparation. Figure 5 compares performance with a simple test mix for 1.9-um Titan C18 and two popular sub-2-µm porous C18 columns under reversed-phase conditions. Higher column efficiency was observed for Titan than traditional porous silica C18 particles with smaller average size and broader PSD. The same high-performance UHPLC instrument was employed for testing.
Independent studies by Gritti and Guiochon12,13 confirmed that Titan C18 silica columns show reduced plate heights as low as 1.7 and compare very favorably in efficiency and permeability to core-type particles; however, the authors cited possible reasons for such high performance other than narrow PSD.
Figure 5 ‒ High chromatographic performance of monodisperse 1.9-µm Titan C18 compared with two broader-distribution sub-2-µm porous C18 columns. Sample: uracil, diazepam, toluene, naphthalene, biphenyl; columns: 50 × 3.0 mm; mobile phase: 60% acetonitrile/40% water; temp: 35 °C, flow rate: 0.9 mL/min (4 mm/sec); detection: 254 nm. New commercial columns were tested as received.
Figure 6 ‒ High-speed separations showing greater retention of 1.9-µm porous Titan C18 column compared to 1.7-µm core-type C18 column for benzodiazepines and metabolites by gradient LC/MS. Columns: 5 cm × 3.0 mm; mobile phase: a) 0.1% formic acid in 95:5, water:acetonitrile; b) 0.1% formic acid in 5:95, water:acetonitrile; gradient: 35% B to 60% B in 1 min; 60% B held for 0.5 min; flow rate: 0.6 mL/min; column temp.: 35 °C; detector: MS-TOF, ESI+.A recent paper by Felinger14 strongly supported narrow PSD as an important advantage for solid-core particles over totally porous particles with broader PSD. A second paper by Gritti and Guiochon15 confirmed higher performance for Titan C18 silica particles having different pores of 80 Å and 120 Å and described when using larger pores should be advantageous.
Current HPLC column platforms from Supelco that employ monodisperse silica particles are summarized in Table 1; silica substrates are chemically modified with a range of useful column selectivities. Fully porous Titan C18 is compared to commercial core-type silica C18 in Figure 6 for separating benzodiazepines and metabolites under gradient LC-MS conditions; as expected, slightly higher retention and equivalent selectivity were observed.
Table 1 ‒ Supelco silica platforms with monodisperse particles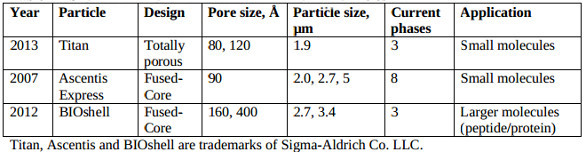
Summary and conclusions
Analytical HPLC/UHPLC columns filled with both porous and core-type particles should continue to improve in efficiency and ruggedness to address the demand for higher sample throughput. Analysts will eventually become less concerned about the type of particle inside as long as the column demonstrates stable, reproducible separation performance. The desire to improve column performance, increase column lifetime and minimize particle waste should continue to drive the production of silica particles toward narrower particle-size distribution. Recent intense development has greatly improved the efficiency of modern spherical silica particles; however, development of interesting new phases has slowed down during this period. Now that new particle designs are becoming widely accepted and readily available, research should shift toward developing new stationary phases to meet the challenge of increasingly complex separations. Selective new phases should be added to proven ones, especially for high-speed resolution of closely related species. The future should also see more use of elevated temperature and multidimensional column techniques in HPLC and UHPLC to solve the most difficult separation problems.
Acknowledgments
The efforts of the large Supelco team who developed the Ecoporous process for synthesizing monodisperse Titan particles are acknowledged and greatly appreciated. Regular communication and support from Drs. Jack Kirkland, Joseph DeStefano, Stephanie Schuster and others at AMT concerning Fused-Core particle technology from 2006 until the present were valuable as well. The assistance and column performance evaluations of academic collaborators Professors Luigi Mondello and Paola Dugo at the University of Messina and Francesco Gasparrini at Sapienza University of Rome are also appreciated. Column evaluations and discussions with Professors Gert Desmet and Ken Broeckhoven, Free University of Brussels, and Professors Fabrice Gritti and Georges Guiochon, University of Tennessee, were very valuable.
References
- Knox, J.H. and Saleem, M. Kinetic conditions for optimum speed and resolution in column chromatography. J. Chromatogr. Sci. 1969, 7, 614‒22.
- Nguyen, D.; Guillarme, D. et al. Review: fast analysis in liquid chromatography using small particles and high pressure. J. Sep. Sci. 2006, 29, 1836‒48.
- Schwartz, M. Ultra performance liquid chromatography (UPLC): an introduction. Special Issue of LC GC May 2005, 8‒14.
- Kirkland, J.J.; Langlois, T.J. et al. Fused-core particles for HPLC columns. Am. Lab. Feb 2007, 39, 18‒21.
- Chester, T. L. Sub-2-micron performance with a conventional HPLC instrument using 2.7-micron Fused-Core particles. Am. Lab. Mar 2009, 41, 11‒15.
- Giddings, J.C. Dynamics of Chromatography. Marcel Dekker, Inc.: New York, NY, 1965, Chapter 2.
- Knox, J.H. Band dispersion in chromatography—a new view of the A term. J. Chromatogr. A 1999, 831, 3–15.
- Knox, J.H. A universal expression for bandspreading in the mobile zone. J. Chromatogr. A 2002, 960, 7–18.
- Henry, R.A. Review: impact of particle size distribution on HPLC column performance. LC GC 2014 North America Apr 2014, 32(4), 12‒19.
- Cabooter, D.; Fanigliulo, A. et al. Relationship between particle size distribution and chromatographic performance. J. Chromatogr. A 2010, 1217, 7074–81.
- Betz, W.R.; Campbell, W.H. Development of a New Monodisperse Porous Silica for UHPLC. Poster presented at 38th International Symposium on High Performance Liquid Phase Separations and Related Techniques (HPLC 2012), June 16‒21, 2012, Anaheim, Calif.
- Gritti, F.; Bell, D.S. et al. Particle size distribution and column efficiency—ongoing debate revived with 1.9 µm Titan-C18 particles. J. Chromatogr. A 2014, 1355, 179–92.
- Gritti, F. and Guiochon, G. Rationale for optimum efficiency of fully porous Titan-C18 particles. J. Chromatogr. A 2014, 1355, 164–78.
- Horváth, K.; Lukács, D. et al. Effect of particle size distribution on the separation efficiency in liquid chromatography. J. Chromatogr. A 2014, 1361, 203–8.
- Gritti, F. and Guiochon, G. Quantitative impact of mesopore size in Titan-C18 fully porous particles; submitted to J. Chromatogr. A (2014).
- Neue, U.D. HPLC Columns; Wiley-VCH: New York, NY, 1997, p 14.
- Antia, F.D. and Horvath, C. High performance liquid chromatography at elevated temperatures. J. Chromatogr. 1988, 435, 1‒15.
- Henry, R.A. et al. Achieving Maximum Kinetic Performance from UHPLC Columns, Oral Paper, Eastern Analytical Conference, Somerset, NJ, Nov 18, 2014.
Richard A. Henry is a Consultant, State College, Penn., U.S.A. Paul Ross, William R. Betz, Gaurang Parmar and Wayne K. Way ([email protected]) are with Supelco, Div. of Sigma Aldrich, 595 North Harrison St., Bellefonte, PA 16823-0048, U.S.A.; tel.: 814-359-3441/800-247-6628.
AppendixThe importance of van Deemter plotsA van Deemter plot should be employed in chromatography to establish column performance and identify optimum velocity (minimum H, or maximum N) during method development. Final methods usually do not require optimum velocity. In a typical experiment, column efficiency (N) is measured for a given length (L) and converted to plate height (H) or reduced plate height (h). It is usually plotted against flow velocity (u) and broken into terms which have different velocity dependence, as shown in the following simple relationships. H = L/N (1) h = H/dP (2) H = A + B/u + Cu (3) u = L/t0 (4) Time (t0) of an unretained peak may be used to estimate flow velocity; however, useful results for columns of the same dimensions can be obtained by plotting H as a function of flow rate. For more complete discussions of flow velocity and the van Deemter equation, see Knox7,8 and Neue.16 As illustrated in Figures 1 and 2, well-prepared columns with solid-core silica demonstrate much smaller h values and show narrower peaks with more plates/meter than porous silica with similar average particle size (dP) but broader PSD. Each term in Eq. (3) contributes to overall column performance but changes in relative importance with flow velocity. The A term is important to performance at all velocities, the B term dominates at low velocities and the C term dominates at high velocities. The A term describes flow uniformity outside the particles and is smaller for solid-core particles because column beds apparently are more uniform. This was somewhat surprising when core-type columns were introduced, and was attributed to rougher surface, higher particle density and narrower PSD (D90/10 = 1.15). Since the A term does not change significantly with velocity, it remains important at all flow velocities and establishes the overall level of column performance. Routine column operation at high pressure can create bed shifting, disruption of flow uniformity and loss of efficiency. The B term describes longitudinal or axial diffusion that is primarily important while solutes are in the mobile phase and able to diffuse more freely; it decreases with velocity as solutes spend less time in the column. Surprisingly, this term is also smaller for core-type particles because the presence of a solid core apparently restricts axial diffusion by reducing internal column volume and opportunities available for free diffusion. While early textbooks have suggested to ignore the B term in HPLC because of slow axial solute diffusion in liquids, this approximation is unrealistic for modern, small-particle columns. The A term has become so small for new particles that the B term may be significant at all flow velocities; and its importance will increase at elevated column temperatures. The C term increases with flow velocity and describes rate of mass transfer between flowing mobile phase and stagnant regions within and around particles. As expected, radial mass transfer is faster for solid-core particles because solutes must travel shorter distances inside the particle to interact and separate. While both porous and core-type particles deliver high performance for small molecules (10,000 MW), having large diffusion coefficients, advantages for solid-core particles with short diffusion paths begin to show when high mobile phase velocities drive high sample throughput. For large molecules (>10,000 MW), the C term becomes critically important and speed-limiting under all flow and viscosity conditions, shifting the advantage further toward a core-type particle. It may be possible to reduce this performance gap between core-type and porous particles for large molecules by operating at elevated temperatures17 to decrease viscosity and increase diffusion rates. The practical use of higher temperature in UHPLC experiments requires more study, however, because any performance gains from faster radial diffusion (smaller C term) may be offset by performance losses from faster axial diffusion (larger B term), especially in the UHPLC experiment where the B term has greater significance.18 Improvements in instrument design may be required before elevated temperature operation becomes a precise tool in UHPLC. Complexity exists for HPLC systems because heater designs and instrument flow paths are not standardized, making results potentially difficult to reproduce between instruments compared to gas chromatography. Further complexity occurs for small particles when UHPLC columns are operated at high flows and pressures because frictional heating and poor thermal equilibration can interfere with flow uniformity and cause performance loss in the important A term. Changes in selectivity may also occur. Frictional heating in UHPLC increases in importance with larger column diameters. Kinetic studies are often employed in academic laboratories; however, h-u plots should be used in industry during HPLC method development and optimization since solutes with different chemical structures often behave differently with mobile phase velocity. When samples contain solutes that exhibit different curve shapes based upon experimental evidence as shown in Figure 2, a compromise in optimum velocity is required. In reversed-phase HPLC, polar solutes generally exhibit curves with smaller B terms and larger C terms, which shifts optimum velocity to lower values and can limit separation speed. |