For the application of induced pluripotent stem cells (iPSCs) and human embryonic stem cells (hESCs) to regenerative medicine and drug discovery research, it is crucial to characterize the identity and potency status of these pluripotent stem cells by measuring molecular markers during cultivation. Although iPSCs share many phenotypic, morphological, functional, and molecular similarities with hESCs, it has become clear that the differences may be equally numerous.1 Specifically, populations of hESCs and iPSCs can exhibit heterogeneity in epigenetic markers, expression of somatic genes, and specific responses to cell fate modulators.1,2
These variations, compounded by potential inefficiencies in the reprogramming process leading to a continuum of partially reprogrammed cells, can introduce variations in cell-based assays and other studies based on pluripotent stem cells. Profiling larger numbers of biomarkers—thereby gaining more accurate, higher-resolution snapshots of hESCs, iPSCs, cancer stem cells, or other pluripotent stem cells—may help provide more consistent, interpretable results from studies of these complex cell populations.
Commonly, flow cytometry and PCR techniques are used to observe the expression of pluripotency markers. Several fluorescently labeled antibodies are commercially available for immunofluorescence, immunocytochemistry, and flow cytometry studies. Quantitative reversed phase-PCR (RT-PCR) has proven to be a robust method for quantitating RNA levels corresponding to pluripotency genes, and multiplex RT-PCR kits are available for assessing the expression of multiple pluripotency genes per sample.3 However, given that mRNA levels do not necessarily correlate with protein levels,4 a complete picture of pluripotency can only be obtained by measuring numerous protein, as well as nucleic acid, stem cell markers.
Recently, more sophisticated experimental and computational methods have emerged to more thoroughly characterize ESCs and iPSCs. These methods include analyses of the proteome, phosphoproteome, and DNA methylation profile.2,5,6 These analyses are informative but can be time consuming and can require specialized expertise.
Multiplexed bead-based immunoassays for detection of pluripotency marker proteins
This article describes novel, multiplexed bead-based immunoassays, which provide a simpler, more accurate way to detect a larger number of pluripotency marker proteins in a single measurement. Based on Luminex xMAP® technology (Luminex Corp. [Austin, TX] and EMD Millipore [Billerica, MA]), these bead-based assays provide fast feedback on the potency status of a cell or tissue culture by measuring protein expression levels in cell lysates.
Figure 1 – Pluripotency markers detected using two bead-based multianalyte assay panels.Two multianalyte bead-based immunoassay panels were developed to monitor stem cell pluripotency (Figure 1). The first, a 4-plex panel, consisted of assays for the transcription factors Oct 3/4, Sox2, Nanog, and c-Myc. Oct 3/4, Sox2, and Nanog play roles in the maintenance of pluripotency and self-renewal in stem cells.7–9 The transcription factor c-Myc is involved in apoptosis, cell differentiation, and cell proliferation.10
The second panel, a 7-plex panel, consists of assays to measure the concentrations of additional pluripotency-related markers, including a transcription factor (KLF4), a translational regulator (LIN-28), transmembrane proteins (E-Cadherin and EpCam), and cell surface glycostructures (TRA-1-60 and TRA-1-81). The zinc finger-containing transcription factor KLF4 has regulatory functions in cell growth, proliferation, differentiation, and embryogenesis. It was one of the factors for iPSC reprogramming used by Takahashi and Yamanaka in 2006.11
The translational enhancer LIN-28 contributes to stem cell pluripotency by possibly blocking miRNA-mediated differentiation.12 The transmembrane proteins E-Cadherin and EpCam are adhesion molecules. E-Cadherin is a calcium-dependent transmembrane protein influencing pluripotency and self-renewal signaling pathways in ES and iPS cells.13 EpCam is a hemophilic, calcium-independent adhesion molecule that is expressed in undifferentiated hESCs and is localized to Oct4-positive pluripotent cells.14,15 TRA-1-60 and TRA-1-81 are glycan modifications of the membrane protein podocalyxin and are expressed on ES cells.16,17 Finally, SSEA1 is the stage-specific embryonic antigen-1 that is expressed in mouse ES cells18 but repressed in human ES cells.19 SSEA1 was included as a negative marker of pluripotency in the panel.
Materials and methods
Cell culture and reprogramming
MCF-7, Ntera2 cl.D1, HeLa, and Jurkat cells were cultured according to ATCC® guidelines in recommended media. Cells were grown to approximately 85% confluence. FibroGRO™ Xeno-Free Human Foreskin Fibroblasts (passage 6 HFF, EMD Millipore) were cultured in FibroGRO LS Complete Medium and reprogrammed according to the STEMCCA™ Cre-Excisable Constitutive Polycistronic (OKSM) Lentivirus Reprogramming Kit (EMD Millipore) protocol as previously described.20 iPS cells were cultured in HEScGRO® Medium for Human ES Cell Culture (EMD Millipore).
Neural differentiation of Ntera2 cl.D1 cells
Ntera2 cl.D1 teratocarcinoma cells were cultured under differentiation and selection conditions in order to produce differentiated cells with neural properties.21 Cells were initially seeded on poly-D-lysine-coated T75 flasks at a density of 3 × 105 cells/flask. After two days’ rest, the medium was changed to medium supplemented with 10 μM all-trans retinoic acid (ATRA, Sigma, St. Louis, MO). This treatment was continued for four weeks (medium changed twice a week, differentiation stage). After four weeks, the medium supplement was changed from ATRA to 5 μM cytosine β-D-arabinose (Sigma), and cells were cultured with this supplement for an additional two weeks (medium changed once a week, selection stage).
Throughout differentiation and selection, undifferentiated cells were removed from the bottom of the flask while differentiated cells grew into a monolayer. Cells were examined using phase contrast microscopy (100-fold magnification, Axiovert® 40, Carl Zeiss, Oberkochen, Germany). Replicate flasks were harvested using Trypsin-EDTA (Life Technologies, Carlsbad, CA) at weeks 0, 3, 4, and 6.
Cell lysate preparation
Cells were washed with ice-cold phosphate-buffered saline. MILLIPLEX® MAP Cell Signaling Lysis Buffer (1×, EMD Millipore) with freshly added protease inhibitors was added to cells (1 mL per 1 × 107 cells). The suspension was rocked for 10–15 min at 4 °C. The lysates were then filtered through a 0.1-μm membrane filter and total protein was quantified using a BCA assay.
Development of bead-based immunoassays
Bead-based multianalyte immunoassay panels (MILLIPLEX MAP Human Stem Cell Pluripotency Magnetic Bead Kits 1 and 2, EMD Millipore) were developed by conjugating specific capture antibodies to MagPlex® microsphere beads purchased from Luminex Corp. Each set of beads is distinguished by different ratios of two internal dyes, yielding a unique fluorescent signature to each bead set. For initial validation of the bead-based assays, immunoprecipitation of the analytes was performed with capture beads and detected by Western blotting with the biotinylated detection antibodies in control cell lines. Cross-reactivity tests were performed using capture beads in multiplex by IP/Western blotting and individual detection antibodies. All validation results were previously reported.22
Multiplexed bead-based assays
Lysate samples were adjusted to a protein concentration of 2 mg total protein/mL lysate and assayed in 96-well plates at a concentration of 20 μg total protein/well using the protocol outlined in Table 1.
Table 1 – Protocol for MILLIPLEX MAP human pluripotency magnetic bead panels*
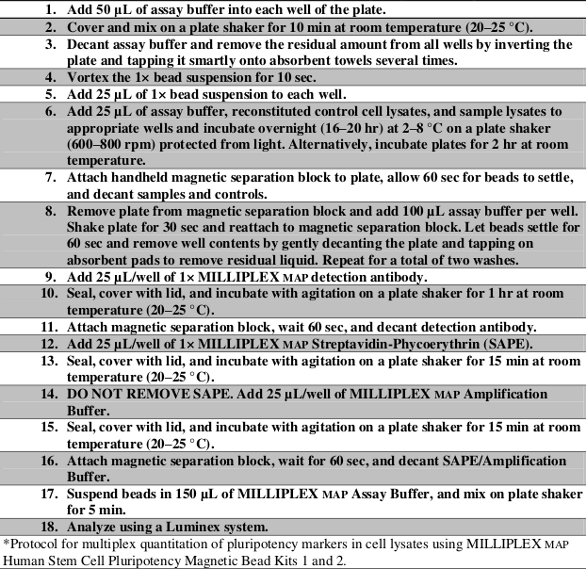
Flow cytometry analysis
Flow cytometry analysis was performed according to the product instructions provided in the FlowCellect® Human iPS Cell Characterization Kit (EMD Millipore). Human iPS stem cell and human foreskin fibroblast cultures were dislodged from a tissue culture plate using a mild enzyme (Accutase™ reagent, EMD Millipore) and placed in suspension in a 96-well plate (100,000 cells/well). Cells were fixed for 20 min, followed by a 10-min permeabilization step. Using a working antibody cocktail solution containing fluorophore-conjugated antibodies to TRA-1-60, Oct4, SSEA1, and SSEA4, cells were stained for 30 min on ice in the dark. Following staining, cells were washed once in 1× assay buffer and resuspended in 200 mL 1× assay buffer in preparation for sample acquisition. Samples were assayed on the guava easyCyte™ 8HT benchtop flow cytometer (EMD Millipore), and analysis was performed using the InCyte™ software module (EMD Millipore).
Results and discussion
The authors demonstrated the use of multiplex bead-based immunoassays to examine different sets of protein biomarkers that indicate the potency of stem cells to quickly and accurately distinguish pluripotent cells from multipotent or differentiating cells. First, multiplex assays were used to monitor the differentiation of pluripotent teratocarcinoma cells into differentiated cells with neural properties. Ntera2 cl.D1 cells treated with retinoic acid (ATRA) followed by mitotic inhibitors (such as cytosine arabinoside) have been shown to generate over 95% pure cultures of neuronal cells that express many neural-specific markers.23
For this study, Ntera2 cl.D1 cells were subjected to four weeks of differentiation, followed by two weeks of treatment with mitotic inhibitor and analyzed at weeks 0, 3, 4, and 6. As previously reported, undifferentiated Ntera2 cl.D1 cells appeared as individual, flattened, adherent cells; after six weeks of differentiation and inhibition of mitosis, cells formed round clumps (Figure 2a). Analysis of the stem cell markers in the same cultures showed a decrease in pluripotency-associated markers, including Oct4, Sox2, Nanog, LIN-28, TRA-1-60, and TRA-1-81, after three weeks of differentiation. The decreases became more marked over time (Figure 2b and c). EpCam expression also decreased significantly with respect to increased cellular differentiation. As an adhesion molecule, it may play a role in the altered morphology of neural-differentiated Ntera2 cl.D1 cells.
Figure 2 – Differentiation of teratocarcinoma cells toward a neural lineage is marked by changes in morphology and marker expression. Compared to undifferentiated Ntera2 cl.D1 cells, cells subjected to differentiation with ATRA and six weeks of selection formed round clumps, as expected (a). Time course of protein marker expression (b, c) showed decreases in expression of pluripotency markers after three weeks of differentiation. Error bars represent standard deviation.The ability of this multiplex assay to simultaneously quantify multiple intracellular and cell surface proteins in a single measurement, in a single sample, provided a convenient method for monitoring protein expression changes occurring in conjunction with morphological changes. Further studies can be envisioned that involve the simultaneous monitoring of cell signaling events to gain deeper insights into the molecular mechanisms of neurite outgrowth and other neural phenotypes.
Bead-based multiplex assays were also used to monitor the generation of iPSCs from human foreskin fibroblasts using a polycistronic, excisable lentiviral vector (Figure 3a and b). Expression of Oct 3/4, Sox2, and Nanog as well as the presence of E-Cadherin, EpCam, KLF4, LIN-28, TRA-1-60, and TRA-1-81 could be observed after reprogramming, indicating that the examined cell culture was indeed pluripotent. Almost all pluripotency marker analytes showed a 57–177 fold increase in expression after reprogramming. The data were consistent with the expression profiles of these markers in flow cytometry experiments (Figure 3c). Pluripotency-associated transcription factor KLF4 was expressed in HFF; on the other hand, the negative marker SSEA1 was not expressed in HFFs or in iPSCs. The control marker c-Myc was expressed at detectable levels in all cells.
Figure 3 – IPSCs show upregulation of pluripotency markers in both multiplex assays of cell lysates as well as flow cytometry. Differentiated human foreskin fibroblasts were reprogrammed by transduction with the STEMCCA Cre-Excisable Constitutive Polycistronic (OKSM) Lentivirus Reprogramming Kit, resulting in hSTEMCCA iPSC and virus-excised hSTEMCCA iPSC. a) HFF and iPS cells were assayed with the 4-plex MILLIPLEX map Human Stem Cell Pluripotency Kit 1 using Ntera2cl.D1 and HeLa as control cell lines. b) HFF and iPS cells were also analyzed with the 7-plex MILLIPLEX mapHuman Stem Cell Pluripotency Kit 2 using Jurkat, MCF-7, and Ntera2 cl.D1 as controls. c) The markers Oct 4, TRA-1-60, and SSEA1 were measured using FlowCellect Human ESC (TRA-1-60) Surface Marker Characterization and Nucleus Marker Characterization Kits. The histograms of the stained HFF control cell lines were overlaid with the histograms of the virus-excised iPSC generated thereof. Error bars in (a) and (b) represent standard deviation.Conclusion
As shown by the authors’ data, bead-based multiplex assays can be successfully used to analyze a wide number of protein markers that are needed to characterize the potency of a stem cell. Intracellular proteins, transmembrane proteins, as well as glycostructures serving as pluripotency markers could be detected in the presented multiplex assays. Compared to traditional techniques, bead-based multiplex assays enable the analysis of a great number of pluripotency markers in a single measurement, saving valuable time and resources, while serving as a fast and reliable complementary method for conventional flow cytometry analyses. The speed and ease of the measurements, combined with their quantitative, multiparametric nature, may facilitate the correlation of marker expression profile to differentiation/pluripotency status, enabling comparisons between cell cultures, from experiment to experiment, and from one laboratory to another. Thus, these assays may increase the consistency and reproducibility of stem cell models for research and therapeutic development.
References
- Plath, K.; Lowry, W.E. Progress in understanding reprogramming to the induced pluripotent state. Nat. Rev. Genetics 2011, 12(4), 253–65.
- Wang, A.; Du, Y.; et al. A quantitative system for discriminating induced pluripotent stem cells, embryonic stem cells and somatic cells. PLoS One 2013, 8(2), e56095.
- Ruff, D.; Lieu, P.T. Profiling stem cells using quantitative PCR protein assays. Meth. Molec. Biol. 2013, 997, 225–36.
- Ghazalpour, A.; Bennett, B. et al. Comparative analysis of proteome and transcriptome variation in mouse. PLoS Genetics 2011, 7(6), e1001393.
- Phanstiel, D.H.; Brumbaugh, J. et al. 2011. Proteomic and phosphoproteomic comparison of human ES and iPS cells. Nat. Meth. 2011, 8(10), 821–7.
- Pera, M.F. The proteomes of native and induced pluripotent stem cells. Nat. Meth. 2011, 8(10), 807–8.
- Nichols, J.; Zevnik, B. et al. Formation of pluripotent stem cells in the mammalian embryo depends on the POU transcription factor Oct4. Cell 1998, 95(3), 379–91.
- Tomioka, M.; Nishimoto, M. et al. Identification of Sox‐2 regulatory region which is under the control of Oct‐3/4–Sox‐2 complex. Nucl. Acids Res. 2002, 30(14), 3202–13.
- Pan, G.; Thomson, J. Nanog and transcriptional networks in embryonic stem cell pluripotency. Cell Res. 2007, 17, 42–9.
- Gandarillas, A.; Watt, F. c-Myc promotes differentiation of human epidermal stem cells. Genes and Development 1997, 11, 2869–82.
- Takahashi, K.; Yamanaka, S. Induction of pluripotent stem cells from mouse embryonic and adult fibroblast cultures by defined factors. Cell 2006; 126(4), 663–76.
- Viswanathan, S.R.; Daley, G.Q. et al. Selective blockade of microRNA processing by Lin28. Science 2008, 320(5872), 97–100.
- Soncin, F.; Mohamet, L. et al. E-cadherin acts as a regulator of transcripts associated with a wide range of cellular processes in mouse embryonic stem cells. PLoS ONE 2011, 6(7), e21463.
- Litvinov, S.V.; Velders, M.P. et al. Ep-CAM: a human epithelial antigen is a homophilic cell-cell adhesion molecule. J. Cell Biol. 1994, 125(2), 437–46.
- Ng, V.Y.; Ang, S.N. et al. Characterization of epithelial cell adhesion molecule as a surface marker on undifferentiated human embryonic stem cells. Stem Cells 2010, 28(1), 29–35.
- Schopperle, W.; DeWolf, W. The TRA-1-60 and TRA-1-81 human pluripotent stem cell markers are expressed on podocalyxin in embryonal carcinoma. Stem Cells 2007, 25(3), 723–30.
- Thomson, J.A.; Itskovitz-Eldor, J. et al. Embryonic stem cell lines derived from human blastocysts. Science 1998, 282(5391), 1145–7.
- Ozawa, M.; Muramatsu, T. et al. SSEA-1, a stage-specific embryonic antigen of the mouse, is carried by the glycoprotein-bound large carbohydrate in embryonal carcinoma cells. Cell Differentiation 1985, 16(3), 169–73.
- Draper, J.S.; Pigott, C. et al. Surface antigens of human embryonic stem cells: changes upon differentiation in culture. J. Anat. 2002, 200, 249–58.
- Sommer, C.A.; Sommer, A.G. et al. Excision of reprogramming transgenes improves the differentiation potential of iPS cells generated with a single excisable vector. Stem Cells 2010, 28(1), 64–74.
- Langlois, A.; Duval, D. Differentiation of the human NT2 cells into neurons and glia. Meth. Cell Sci. 1997, 19, 213–19.
- http://www.millipore.com/techpublications/tech1/an5528en00.
- Pleasure, S.J.; Page, C. et al. Pure, postmitotic, polarized human neurons derived from NTera 2 cells provide a system for expressing exogenous proteins in terminally differentiated neurons. J. Neurosci. 1992, 12(5), 1802–15.
The authors are with EMD Millipore Corp., 14 Research Park Dr., St. Charles, MO 63304, U.S.A.; tel.: 866-441-8400; e-mail: [email protected]. The authors would like to thank Mark Santos, Vi Chu, Reeti Maheshwari, Yasmin Trumpfheller, Alexandra Krog, Chandreyee Das, and Danielle Pepin for their various contributions to this work.