Despite its widespread applications, infrared microspectroscopy has fundamental spatial resolution limits set by both the laws of optics and practical design constraints. Fourier transform infrared spectroscopy is generally limited to a spatial resolution of three times the wavelength of the IR radiation. With attenuated total reflection (ATR), it may be possible to achieve resolution approaching the wavelength of the radiation. The technique described in this article breaks new resolution limits, providing both chemical and physical property data on the scale of ~200 nm and beyond.
From microspectroscopy to nanospectroscopy
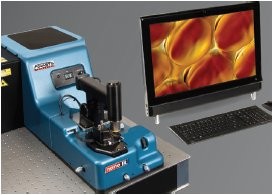
Figure 1 - nanoIR system.
The nano IR™ system (Figure 1 ) (Anasys Instruments, Santa Barbara, CA) breaks through the resolution limits in conventional IR spectroscopy by using the tip of an atomic force microscope (AFM) probe to measure infrared absorption. The sample is illuminated by a tunable IR source. When IR radiation is absorbed by a region of the sample, the region heats up. The heat generates a rapid thermal expansion pulse that can be detected by the AFM cantilever tip. In contrast to “far-field” optical diffraction, the absorbed radiation is measured by the tip in the extreme near field.
Atomic force microscopy successfully addresses problems in basic nanoscale research as well as applied problems in materials science and engineering. The AFM has also been widely credited with enabling multibillion-dollar research investments in nanoscience and nanotechnology. A clear gap in AFM capabilities, however, is the ability to chemically characterize regions of the sample. In fact, the capacity to identify material under the tip of an AFM has been identified as one of the major goals for probe microscopy.
While AFM can measure the mechanical, electrical, magnetic, and thermal properties of materials, it lacks the ability to chemically characterize unknown materials. In contrast, the nanoIR instrument employs infrared spectroscopy to provide chemical analysis of samples on the submicron-length scale. Infrared spectroscopy measures the wavelength-dependent absorption of radiation that results from excitations of specific molecular vibrations. The resulting absorption spectra provide rich information about the chemical content of material under the AFM tip.
The resolution of conventional FTIR microspectroscopy is constrained by a fundamental physical limit called the diffraction limit. The diffraction limit prevents spatial resolution from exceeding some multiple of the wavelength of light being used. As a rule of thumb, these limits are roughly three times the wavelength for conventional transmission measurements and about the wavelength for more sophisticated measurements such as ATR. In the C–H region, this corresponds to approximately 3–10 µm. In comparison, the nanoIR instrument has achieved spatial resolution at scales as low as 200 nm.
Principle of operation
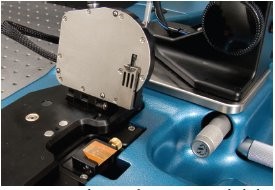
Figure 2- Close-up of ZnSe prism in which the samples are mounted for the nanoIR.
The nanoIR system applies the patent-pending technology of infrared nanospectroscopy, or AFMIR, which was pioneered by Dr. Alexandre Dazzi of the Laboratoire de Chimie Physique, CLIO, Université Paris-Sud (Orsay, France). The nanoIR system uses a pulsed, tunable IR source to excite molecular absorption in a sample that has been mounted on a ZnSe prism (see Figure 2). Samples are prepared in one of two ways. For many samples, ultramicrotomy is used to cut sections with thicknesses between 100 and 1000 nm (Figure 3). These are then transferred to the prism surface. In other sample preparations, it is possible to cast thin films from solution directly onto the prism.
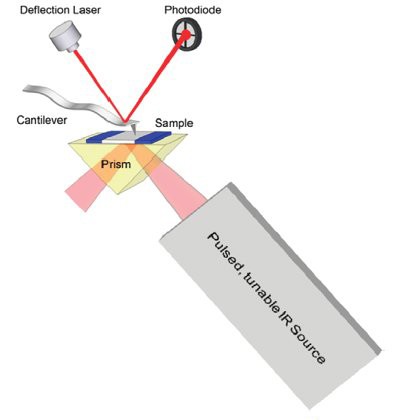
Figure 3 - Schematic showing the optical path of the IR source and the AFM beam deflection detection.
The IR beam illuminates the sample by total internal reflection similar to conventional ATR spectroscopy. As the sample absorbs radiation, it heats up, leading to rapid thermal expansion that excites resonant oscillations of the cantilever, which is detected using the standard AFM photodiode measurement system (see Figure 3). These induced oscillations decay in a characteristic ringdown that can be analyzed via Fourier techniques to extract the amplitudes and frequencies of the oscillations. Then, measuring the amplitudes of the cantilever oscillation as a function of the source wavelength, local absorption spectra are created (Figure 4).
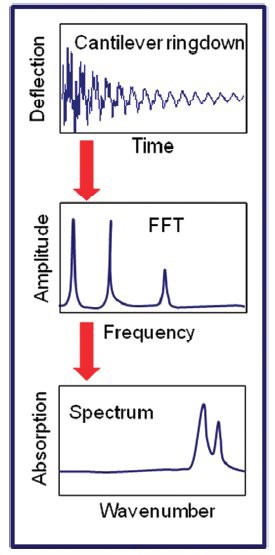
Figure 4 - Schematic demonstrating the cantilever ringdown and generation of the IR spectrum.
The oscillation frequencies of the ringdown are also related to the mechanical stiffness of the sample. Therefore, mechanical properties of a sample can be collected using the contact resonance method to map stiffness variations simultaneously with the topography. With maximum flexibility, the IR source can also be tuned to a single wavelength to simultaneously map surface topography, mechanical properties, and IR absorption in selected absorption bands. Possible areas of application are found in the polymer, materials, and life sciences, offering the potential to produce detailed studies of structure–property correlations.
The ability to combine nanoscale spatial resolution with chemical spectroscopy provides users of nanoIR technology with the tools to quickly survey regions of a sample via AFM and then rapidly acquire high-resolution chemical spectra at selected regions on the sample. The technique also allows additional mechanical and thermal properties measurements with nanoscale resolution.
Researchers can harness the full power of mid-IR spectroscopy with the system. The nanoIR’s infrared source, designed using proprietary technology, is continuously tunable from 3600 to 1200 cm–1. This range covers a major portion of the mid-IR, including important CH, NH, and CO bands, as well as carbonyl and amide I/II bands. Polymer spectra acquired with the system have demonstrated good correlation with bulk FTIR spectra. The nanoIR software also allows researchers to export nanoscale IR absorption spectra to standard analysis packages (e.g., KnowItAll® software, Bio-Rad, Hercules, CA) to rapidly analyze samples and identify chemical components.
The nanoIR platform also incorporates a measurement technique called nanoscale thermal analysis. This technique makes use of a microfabricated AFM cantilever that incorporates a very small heater into the end of the cantilever, allowing rapid measurements of the transition temperature of the material that is in contact with the apex of the tip of the AFM cantilever. This enables researchers to work at spatial resolutions and sample volumes that are orders of magnitude smaller than traditional thermal analysis measurements to obtain information not available with other techniques.