The extraction of organics from water
is fundamental in environmental,
pharmaceutical, process, food, forensic,
and other laboratories. Nearly all laboratories
that perform organic analyses are
equipped with a battery of separatory funnel (SF) and/or continuous liquid-liquid
extractors (CLLEs) to conduct liquid-liquid
extraction. For environmental laboratories
that conduct liquid-liquid extractions in
high volume on a routine basis, this task
can be a critical element, often a bottleneck
of a laboratory operation. The U.S. EPA
imposes a seven-day holding time on water
sample preparations, imparting a significant
burden on extraction technicians, especially
when there are so often delays in getting
the samples from the field to the laboratory.
If holding times are not met, analysis
results are invalid and the laboratories are
commonly charged with the responsibility
to resample.
Laboratories equipped to do either CLLE
or SF liquid-liquid extractions (for the
environmental laboratory, most commonly
Method 3510C or Method 3520C1) choose
one or the other by considering the advantages
and disadvantages of each. An assessment
is made of available labor and capacity
to extract the number of samples on hand,
meet holding time requirements and data
quality objectives, as well as turnaround
time commitments. SFs are used primarily
because a few sample preparations can be
completed within several hours. However,
SF extraction is extremely labor intensive
and often fraught with problems of precision
and accuracy. Emulsions, rendering the
phase separation difficult and sometimes
impossible, are too frequently observed.
Extraction recovery can be inherently low
for some species. CLLEs are costly and slow,
difficult to clean, readily broken, and subject
to drip rate variation, but require much
less labor. For the extraction of herbicides
from water using a lighter-than-water solvent,
the use of CLLE is precluded, leaving
no choice. The especially cumbersome herbicide
method, with its extra steps, was an
additional driving force behind the development
of the technique described here,
so-named bottle liquid-liquid
extraction
(BLLE, or "Billie").
A simple concept in which water, in original
sample bottles received directly from
the field, is mixed with solvent and turned
at a slow rate was explored and found to be
markedly effective, eliminating the many
problems associated with both SF and
CLLE. The advantages of this new method
are readily recognized by organic analysts
who perform high-volume liquid-liquid
extractions on a routine basis. Most notable
benefits are striking simplicity and economy;
radical reduction of labor, solvent,
and solvent waste; ability to prepare a large
number of samples at once; as well as freedom
from emulsions. Extraction times can
be significantly reduced while precision and
overall quality are improved. There is no
glassware to clean, no elaborate equipment
needed, and virtually no labor required.
The use of chlorinated solvents and other
expensive supplies is sharply curtailed (a "green" technique).
Laboratory control sample (fortified
blank) efficiencies for nearly 200 of
the most important environmentally
regulated semivolatile organic analytes
including herbicides, pesticides,
Method 6252 components, and Appendix
IX3 Method 8270C target analytes
were examined and compared to those
obtained using conventional SF or CLLE
techniques. While the study focuses on
species of environmental interest, extraction
efficiencies for a host of related
chemical species can be readily gleaned.
Also presented, an outcome of hundreds
of studies conducted to validate this
procedure, are little known but distinct
recovery patterns and trends observed
among SF, CLLE, and BLLE, which will
help analysts to improve data quality.
The dramatic effects of starting the semivolatile
extraction at an acidic pH as
opposed to base first are elucidated.
Experimental
Early experiments were conducted by rotating
bottles on two ordinary barbecue rotisseries
spaced a few inches apart mounted on
a wooden substrate. Two-inch sections of
rubber hose were cut and slid over square
rotisserie rods to create a smooth, rotating
surface. Clear, wide-mouth 1-L glass jars
were filled approx. 90% full with tap water
and fortified with several drops of a 0.1%
solution of methyl yellow (p-dimethylaminoazobenzene)
in methanol, followed
by a few drops of 1:1 sulfuric acid. (Methyl
yellow, an Appendix IX target analyte, yellow
in color in basic water and bright red in
acidic conditions, served as a visual indicator
of extraction efficiency.) Forty milliliters of
methylene chloride (DCM) was then added
along with 10 g of rock salt. The jar was
placed horizontally on the rotisserie rack and
turned at approx. 3 rpm for 22 hr. After the
22-hr period, the water layer was completely
clear and the DCM layer was bright yellow.
The experiment was repeated, altering the
initial pH to basic conditions. After 22 hr, the DCM layer was observed to be bright
yellow, while the water layer retained a
yellow tinge.
Encouraged by this crude initial study,
more elaborate experiments were
designed, fortifying deionized, charcoal-filtered
water in 1-L bottles with 50 µg of
a 76-component
mixture of semivolatile
organics (Mega-Mix, catalog no. 31850,
Restek Corp., Bellefonte, PA) containing
acid, neutral, and basic extractables. Traditional
8270C surrogates were also added at
levels customary for laboratories following
environmental procedures. The pH was
brought down to <2 and 40 mL DCM was
added. Following a 22-hr turn at 3 rpm,
the bottle contents were transferred to a
2-L separatory funnel and the DCM was
collected. The water layer was returned to
the bottle, the pH readjusted to >12, and
a second 22-hr turn was performed. The
combined acid/neutral and basic extracts
were concentrated to 1 mL using a Turbovap
apparatus (Zymark Corp., Hopkinton,
MA) prior to analysis by the conventional
environmental 8270C method. All
components were recovered, most with
75% or greater recovery. Phenol, pyridine,
and N-nitrosodimethylamine were low,
however, recovering only at about the
10% level.
Additional studies were undertaken to
improve the recovery of the phenol and
amines. The ionic strength of the water
was increased by adding sodium chloride,
and the effect of intermixing diethyl
ether and other solvents with the DCM
was explored. Extracting with a 60/40-mL
mix of DCM/diethyl ether in place of pure
DCM was found to markedly enhance the
recovery for phenol. Adding sodium chloride
to the water (250 g per 1000 mL) significantly
improved the recovery for the
pyridine and other amines.
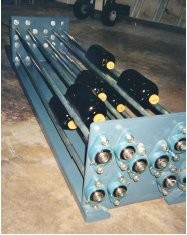
Figure 1 - Photograph of prototype BLLE bottle rotation device.
A heavy-duty turning apparatus was constructed using five sets of 6-ft-long galvanized rods mounted on a rack driven by an electric motor, sprockets, and chain (Figure 1). The device was engineered to turn at triple the earlier experiment rate (12 rpm) as well as to enable 30 1-L bottles to be spun at once. With this apparatus, scores of additional experiments were conducted over years of time to examine the effects of the faster spin, varied durations of spin, alternate mixtures of salt, ether, as well as reversing the extractions by initializing with base rather than acid (Method 625). A vented stopcock bottle cap adapter was designed (Figure 2) to allow the solvent to be removed directly from the bottle, thereby eliminating the need to transfer to a separatory funnel.
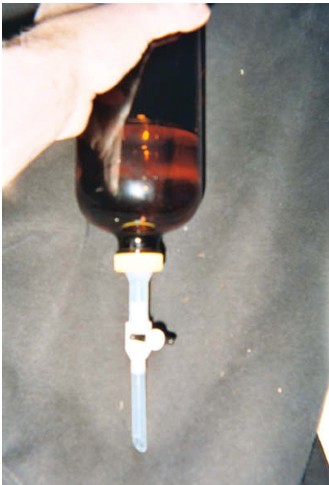
Figure 2 - Photograph of bottle cap attachment enabling phase separations to be conducted directly in bottles.
Using the new rotisserie, the following procedures were optimized and are offered here for use:
Procedure A: DCM/ether—Generally semivolatile acid and base-neutral
extractables (Environmental Methods 8270 and 625, specifically)
Step 1: Remove the sample bottle from refrigerated storage and allow to equilibrate to room temperature.
Step 2: Shake the bottle briefly to homogenize the sample. Discard up
to 250 mL and mark the water level meniscus on the bottle for subsequent
accurate volume measurement.
Step 3: Fortify the sample with surrogates and quality control (QC) samples with appropriate solutions. Shake to mix.
Step
4: Adjust the pH of the sample with approx. 3 mL of 12N sulfuric acid.
Check that pH is ≤2 with wide-range pH paper. (A lesser amount of a
stronger acid solution may be substituted.)
Step 5: Add 100 mL of a
60/40 mix of DCM/diethyl ether (BHT-preserved ethyl ether used for this
study; methanol-preserved likely as good).
Step 6: Cap bottle, place
horizontally on rotation rack, and spin for 12 hr at 12 rpm. (Because
the spinning is gentle, there is no pressure buildup, and venting is not
necessary.)
Step 7: Attach an adapter and stopcock to the bottle or
transfer the bottle contents to a conventional separatory funnel to
effect phase separation. Discharge the DCM/ether layer (heavier than
water) into a 200-mL bottle. Add approx. 10 g of sodium sulfate to the
extract and mix.
Step 8: If using a separatory funnel, return the
water to the original bottle and readjust the pH of the water to ≥12
(check with pH paper) using approx. 9 mL of 6N NaOH or a smaller
quantity of 10N NaOH.
Step 9: Add 80 mL of a 50/30 DCM/ethyl ether mix and return to rotisserie for a second 12-hr period.
Step
10: Reattach an adapter and stopcock to the bottle or transfer the
bottle contents to a separatory funnel to effect phase separation.
Combine the DCM/ether layer with the initial DCM/ether extract.
Step
11: Allow the extract to remain in contact with sodium sulfate for at
least 2 hr, mixing periodically. If the sodium sulfate forms clumps, add
additional sodium sulfate until it remains free-flowing. (Adding sodium
sulfate directly to the extract in place of a common practice of
pouring the extract through a funnel or column of sodium sulfate serves
several purposes. The sodium sulfate remains in contact with the extract
for longer periods of time, the successful removal of water is visibly
indicated by an absence of clumps, and much less sodium sulfate is
required.)
Step 12: Drain the extract through a glass fiber filter
and concentrate the extract using conventional evaporative techniques.
Submit for analysis.
Step 13: Recharge the original bottle with tap
water to the original mark and pour into a 1000-mL volumetric flask to
record the exact volume extracted.
Procedure B: Hybrid—Generally semivolatile
acid and base-neutral extractables
(Environmental Methods 8270
and 625, specifically)
Steps 1-7: Identical to Procedure A
Step 8: Returning the water to the original
bottle, add 190 g of sodium chloride and
shake to dissolve.
Step 9: Readjust the pH of the water to ≥12 (check with pH paper) using approx.9 mL of 6N NaOH or a smaller quantity of
10N NaOH.
Step 10: Add 80 mL of DCM and return to
rotisserie for a second 12-hr period.
Steps 11-14: Identical to steps 10-13 of
Procedure A.
Procedure C: Herbicides (Environmental
Methods 8151 and 615 including hydrolysis step)
Step 1: Remove the sample bottle from
refrigerated storage and allow to equilibrate
to room temperature.
Step 2: Shake the bottle briefly to homogenize
the sample. Discard approx. 250 mL
and mark the water level for later measurement.
Step 3: Fortify the sample with surrogate
and batch QC samples with appropriate
solutions.
Step 4: Add 190 g of sodium chloride and
shake to dissolve.
Step 5: Adjust the pH of the sample with
14 mL of 6N NaOH. (Check that pH is =12 with wide-range pH paper.)
Step 6: Cap bottle, place on rotation rack,
and spin for 2 hr at 12 rpm.
Step 7: Add 100 mL DCM to the water in
the bottle and turn for an additional 4 hr.
Step 8: Attach an adapter and stopcock to
the bottle or transfer the bottle contents to
a separatory funnel to effect phase separation.
Discard the DCM layer and return
the water layer to the original bottle.
Readjust the pH of the water to =2 (check
with pH paper) using approx. 14 mL of
12N sulfuric acid.
Step 9: Add 140 mL of diethyl ether
(BHT-preserved required as opposed to
methanol-preserved to avoid quenching
subsequent methylation) and return to the
rotisserie for a 12-hr turn.
Step 10: Reattach an adapter and stopcock
to the bottle or transfer the bottle contents
to a separatory funnel to effect phase separation.
Collect the ether layer (top layer) in
an acid-washed 250-mL bottle containing
approx. 10 g of acidified sodium sulfate.
Step 11: Returning the water to the original
bottle, add an additional 100 mL of
diethyl ether and spin for an additional
12-hr period.
Step 12: Reattach an adapter and stopcock
to the bottle or transfer the bottle contents
to a conventional separatory funnel
to effect phase separation. Add the ether
layer to the original ether extract in the
250-mL bottle containing sodium sulfate.
Step 13: Allow the extract to remain in
contact with sodium sulfate for at least 2
hr, mixing periodically. If the sodium sulfate
forms clumps, add additional sodium
sulfate until it remains free-flowing.
Step 14: Concentrate and derivatize
the extract conventionally and submit
for analysis.
Step 15: Recharge the bottle with tap
water to the original mark and measure the
sample volume using a 1000-mL volumetric
flask.
Results and discussion
Tables 1-3 summarize dozens of laboratory
control sample and method blank analyses.
Control matrices of purified water were
fortified with a methanolic mix of compounds
prior to pH change and solvent
addition. Under the column header "List,"
analytes associated with Method 625, the
Appendix IX Groundwater Monitoring
List, or the Toxicity Characteristic Leaching
Procedure (TCLP) are encoded with
the character 6, A, or T, respectively. In
each table, applicable QC limits obtained
from Method 625 are provided as a frame
of reference. Recovery limits for those
compounds not associated with Method
625 are arbitrarily set to 40-120. Surrogate
recovery limits are those established by
the U.S. EPA Contract Laboratory Program
(CLP) for semivolatile organics. A
composite of the results for a number of
associated method blanks is provided. The
pound sign (#) is used to call attention to
analytes of special interest.
Table 1 - Bottle extraction: Replicate results of four techniques
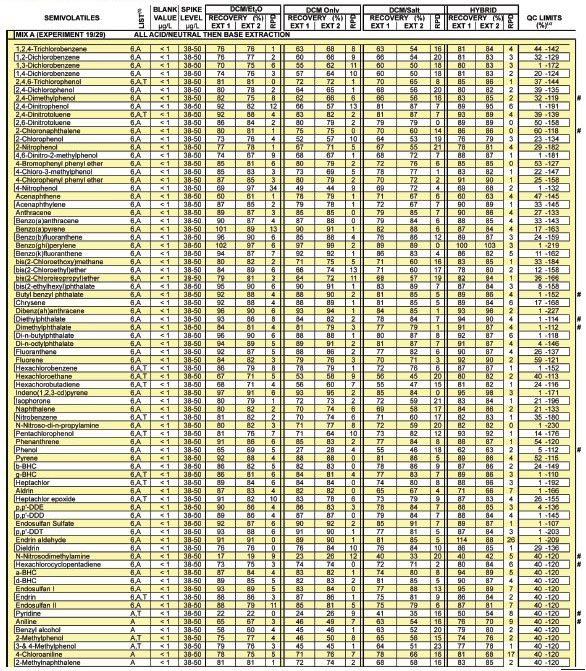
Table 1 - Bottle extraction: Replicate results of four techniques continued
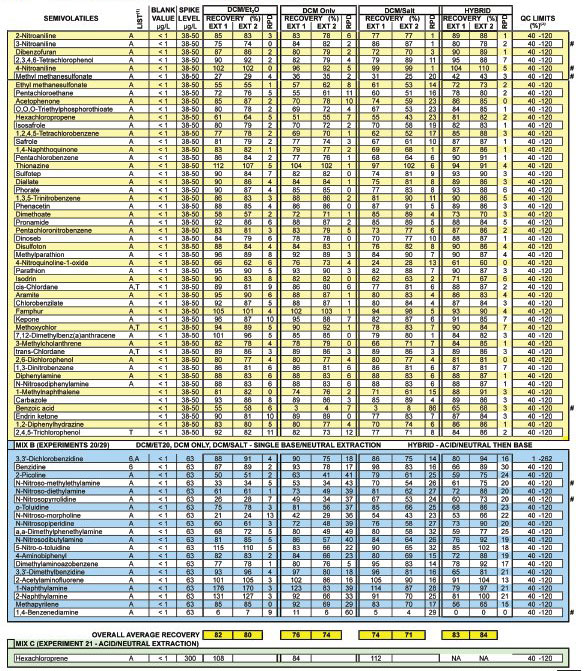
Table 1 - Bottle extraction: Replicate results of four techniques continued
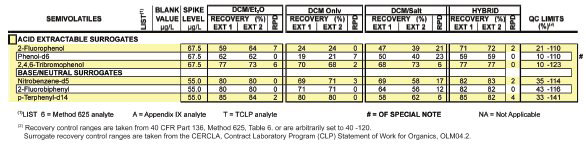