The principles and theory of high-speed capillary chromatography
have been known since the 1960s. To realize the
maximum speed capabilities, it is necessary to use capillaries
with a diameter of approx. 5–50 μm. However, capillary
columns with a diameter smaller than 100 μm are rarely used
because they require very small sample quantities that are
difficult to inject and detect. To overcome this problem,
multicapillary columns (MCCs) composed of a large number
of capillaries were developed.1 Due to much larger surface
and cross-sectional areas, MCCs overcome the flow rate,
volume, and sample capacity limitations associated with
single-capillary columns. MCCs are compatible with standard chromatographic equipment and work with all common
sample sizes and injection techniques. No extensive
modifications of the injector and detector are needed.
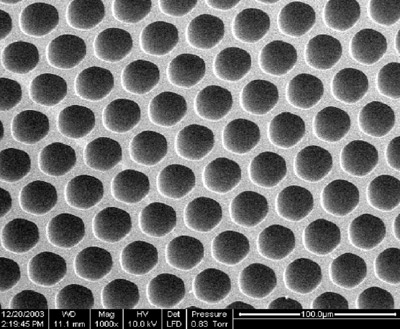
Figure 1 - Cross-section of a multicapillary column with capillary
diameters approx. 20 μm.
A cross-section of an MCC is shown in Figure 1. The capillaries
in an MCC are highly uniform but are not identical,
with a radius dispersion of about 2%. According to
Poiseuille’s law, the mobile phase velocity in wider capillaries
is higher than in smaller capillaries. Obviously, the efficiency
of an MCC cannot be better than that of a single capillary.
Assuming that the stationary phase film thickness in
the capillaries is proportional to the capillary radius in power
a, the height equivalent to a theoretical plate (H) of an
MCC can be expressed by the following equation1:

where HC is the H of an average radius capillary, HO is the H
for a nonsorbing analyte, kC is the retention factor of the
analyte in the capillary of an average radius, and a is a constant
that depends on the method used for the stationary
phase deposition. The MCC efficiency is maximal at a = 3.
Typically, an MCC for GC contains 1000–4000 capillaries of
10–40 μm i.d. in a monolithic glass rod of 1–3 mm o.d. in a
20–100 cm helix. The characteristics of MCCs in comparison
to other types of GC columns are listed in Table 1.
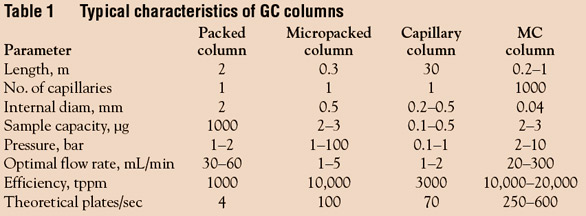
The high sample capacity of an MCC decreases the chance
of overloading the column. Samples with a wide range of
concentration of analytes require large injection volumes for
the accurate detection of the less-concentrated components.
The sample capacity of an MCC with a 0.2-μm stationary
phase film thickness exceeds that of a conventional 0.53-
mm-i.d. capillary column with a 0.25-μm film thickness.When there is no need for extreme sample capacity, an
MCC is also a superior alternative to a packed column.
A van Deemter graph demonstrates the efficiency of a
chromatographic column expressed as height equivalent
to a theoretical plate versus linear velocity of a mobile
phase. The minimal region on the curve represents the
optimal linear velocity that provides the highest efficiency.
MCCs with 40-μm-diam capillaries produce more
theoretical plates per meter than a conventional single-capillary
or packed column, though their short length hinders
their total efficiency. Compared to conventional
single-capillary columns, MCCs have a much wider optimal
mobile phase velocity window on a van Deemter
curve. This allows one to use high flow rates in order to
elute strongly retained analytes, and use low flow rates for
fast-eluting components.
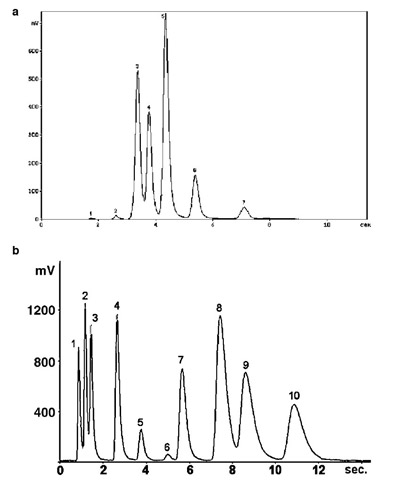
Figure 2 - a) Analysis of industrial solvent by multicapillary gas–liquid chromatography. b) Analysis of C1–C4 hydrocarbons by multicapillary
gas–solid chromatography.
The greatest advantage of MCCs is the speed of analysis.
Separations are achieved approximately ten times faster
than on conventional single-capillary columns. The ultrarapid
analysis of the industrial solvent by gas–liquid chromatography
is shown in Figure 2a. Seven hydrocarbons were
separated in less than 10 sec. Figure 2b illustrates the analysis
of C1–C4 hydrocarbons by gas–solid chromatography. Ten
hydrocarbons were separated in less than 15 sec.
The diffusion of molecules in liquids is much slower than in
gases. For sufficient mass transfer, the diameter of capillary LC
columns should be substantially smaller than that of the capillaries
used in GC. Due to miniscule sizes and sample capacity,
LC capillary columns are not compatible with standard chromatographic equipment and have very limited applications.
Virtually all routine LC separations are conducted on packed columns. The MCCs overcame this limitation and have the
potential to substantially shorten the analysis time of countless
samples in a wide variety of
LC applications.
The separation of the adjacent
chromatographic peaks
is quantitatively expressed by
the resolution factor (RS)
defined as the distance
between the two peak centers
divided by the average
peak width. The resolution
factor can be expressed by
Eq. (2) via the major chromatographic
parameters of
selectivity (α), efficiency (N), and retention (k2) for the
second peak2:
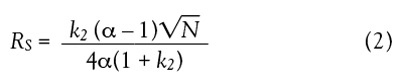
In HPLC, α ≥1.1 is common and k2 = 4–5 is optimal. It
means that the baseline resolution of two analytes (RS
≥1.25) can be achieved on a column having N ≤5000. In
other words, most mixtures can be separated with sufficiently
good resolution on the column, providing a total efficiency
of approx. 5000 theoretical plates.
The manufacture of MCCs for LC is complicated. A stationary
phase, insoluble in common organic and water-organic
solvents, must be deposited on the capillary walls in a manner
that compensates for the polydispersity of the capillaries. At
present, MCCs for LC provide up to 2000 theoretical plates
per column of 15–25 cm length. However, progress in this
area is rapid. MCCs for LC with an efficiency of 5000 theoretical
plates per column will soon be commercially available.
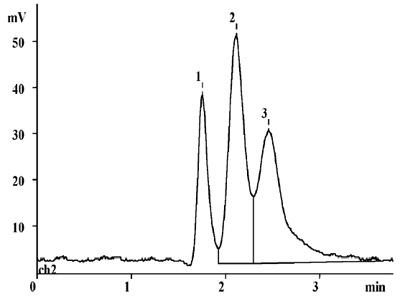
Figure 3 - Separation of 1) uracil, 2) fluorene, and 3) phenanthrene
by multicapillary LC.
MCCs are used for the following applications: fast HPLC separations,
the fractionation of complex mixtures, desalting of
biological samples, solid-phase extraction, and concentration
of samples prior to instrumental analyses. Figure 3 illustrates a
fast separation of a mixture of three organic compounds.
One of the most integral aspects in the preparation of a good
biological sample for further instrumental analysis is the
elimination of impurities. For example, the analysis of
bovine serum albumin digest peptides by matrix-assisted laser desorption ionization mass spectrometry (MALDI-MS)
suffers greatly due to the presence of salts, buffers, and low-molecular-weight organic compounds commonly used in the
preparation of biological samples. MCCs are highly desirable
for quick and effective desalting, purification, and fractionation
of digest peptides, and other biological samples prior to
MALDI-MS and other instrumental analyses.