Proton transfer reaction-mass spectrometry (PTR-MS) is a well-established analytical tool for the measurement of volatile organic compounds (VOCs), and offers real-time detection and quantification of VOCs at trace concentrations. This paper focuses on the measurement of VOCs in biological systems. Both microorganisms and cells, e.g., in the human body, constantly produce a large variety of volatile organic metabolites. Analyzing VOCs in exhaled human breath reveals information about the status of the body. In a similar manner, monitoring the off-gas of fermentations in the biopharmaceutical industry allows microbial activity to be gauged. Undesired compounds (those that are harmful to the human body or impurities in biotechnical processes) can also be tracked in real time using the technique.
A tool for real-time breath analysis
A large variety of VOCs can be found in the human body. Some are exogenous compounds that have been inhaled or ingested, but most are endogenous byproducts of the metabolism process. In the blood–air exchange in the lungs, volatile compounds are released from the blood into the air and are present in exhaled breath in trace amounts. Our knowledge of this process has existed for more than 2000 years: In ancient Greece, physicians used breath analysis as a diagnostic tool. Now we know, for example, that a sweet, fruity breath aroma originates from high levels of acetone in patients with diabetes.1
Breath analysis is an exciting field, yielding a noninvasive diagnostic tool that provides a “window into the body.” Indeed, breath gas analysis of VOCs has become a growing field of research in recent years, with promising potential for medical applications such as the detection of certain diseases, therapy monitoring, and drug testing.2–5
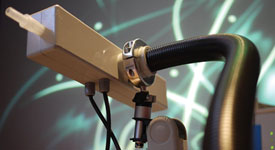
Figure 1 - BET breath sampler.
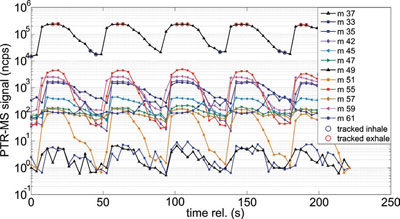
Figure 2 - PTR-MS signals of a real-time breath measurement showing five exhalations into the BET sampler. Here, the gas humidity (blue triangles) is used as a tracer for the room air (green circles) and the exhaled end-tidal air (red circles). In this measurement, 30 m/z in parallel are measured (only 12 are shown).
PTR-MS instruments are ideal tools for breath gas analysis. Their fast response time and high sensitivity allow breath analysis in real time, eliminating the need for sampling containers with storage-induced artifacts, and, importantly, they deliver immediate results.6 One challenge remains: The relevant information is imprinted only in the end-tidal breath fraction, the air that has taken part in the blood-gas exchange. Sampling a full exhalation with high time resolution and selecting the end-tidal fraction using software is possible. However, this phase lasts only a few hundred milliseconds, which is a true challenge even for real-time analysis. A buffered end-tidal (BET) breath gas sampler (Ionimed Analytik GmbH, Innsbruck, Austria) has been developed that extends this end-tidal sampling time to several seconds (Figure 1).7 In the example breath measurement shown in Figure 2, the elongated end-tidal phase can be seen. Detecting compounds with ppt concentrations from a single exhalation is possible. The BET sampler offers further benefits that are essential for artifact-free breath sampling, such as inert and heated inner surfaces to avoid condensation. Clinical requirements, such as disposable mouthpieces with nonreturn valves, are also fulfilled.
The application of PTR-MS in medical research is a fast-growing field, which is demonstrated by a recent focus issue of the Journal for Breath Research.8 In a clinical study, the authors used the PTR-MS to analyze the breath of several hundred patients. In such a screening study, a large spectrum of breath volatiles is measured to look for differences between groups.9,10 Careful analysis of the data involves advanced biostatistical methods to first find the “needle in the haystack” and then to verify that it actually is a needle. As an example, the authors have found two breath markers that can be used to detect a specific histological type of lung cancer. The detection efficiency (area under receiver operating characteristic [ROC] curve) in this case is above 83%.11
Besides the endogenous compounds (the volatile compounds produced by the metabolism itself), smoking has a strong influence on breath composition. The most prominent breath marker for smoking is acetonitrile. The acetonitrile concentration in smokers (between 56 and 110 ppb [the limits of the interquartile range], with a median of 84 ppb) is more than 30 times higher than in nonsmokers (between 1.9 and 3.7 ppb, with a median of 2.5 ppb).9 The concentration in smokers remains elevated even days after smoking the last cigarette. The measurement of exogenous organic compounds in breath, which have been inhaled and accumulated over time, can also be applied to the study of workplace safety of people exposed to hazardous atmospheres.
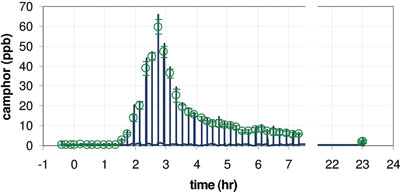
Figure 3 - Pharmacokinetic measurement. A capsule containing camphor is ingested at t = 0. Until t = 1.5 hr, no notable camphor concentration is detected in breath. Then, a sharp increase and a slow decay are observed. Even 23 hr after ingestion a clear signal (1.5 ppb) can be detected.
An exogenous compound can also enter the bloodstream via a different route. Following is an example of a pharmacokinetic study in which the uptake and elimination of a drug in the body is measured. Figure 3 shows a series of breath tests from a single person over the course of 24 hr. At t = 0, the test subject ingested a capsule that contained camphor. The enteric-coated capsule released its contents in the intestinal tract of the test subject, where the camphor is absorbed into the bloodstream and thus becomes visible in the breath. The time delay of approx. 1.5 hr between ingestion and compound release can be clearly observed. Also, the excretion of the compound from the body is evident. Even 23 hr after ingestion, a distinct signal is easily detectable. This demonstrates noninvasive and direct monitoring of blood concentrations by breath analysis. The sampling frequency of >5/hr would be almost inconceivable with invasive blood testing.
Since several hundred volatile metabolic products can be found in the breath, with acetone and isoprene being the most abundant, the usefulness of the direct study of metabolic processes using breath analysis is supported. A specific metabolic process may be difficult to target, since low-molecular-weight metabolites are a product of many different metabolic processes. By administering a metabolic compound, the process of interest can be stimulated. Moreover, using a 13C-isotope-labeled compound will result in labeled metabolites with different molecular mass, which can be distinguished from the natural metabolites by the mass resolution of PTR-MS.
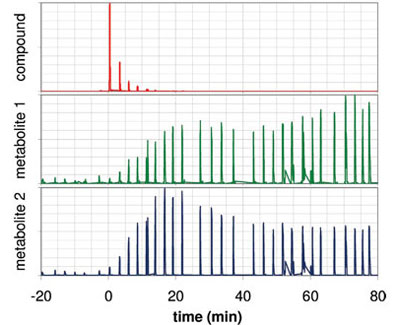
Figure 4 - After ingestion of an isotopically labeled compound, the exhaled concentration of this compound decreases rapidly, while two labeled metabolites appear on different time scales. Each signal has been normalized to the maximum concentration.
Figure 4 demonstrates proof of this principle. The decay of an administered isotopically labeled compound and the appearance of two metabolic products with distinguishable time response is observed. This principle is already well established in breath tests involving 13C-labeled compounds and the measurement of their metabolic degradation to the end product, 13CO2. This approach opens up new possibilities, including free design of the metabolic labeling. By administering a mixture of labeled compounds, several metabolic processes could be probed in a single test.
PTR-MS applications in biotechnology
Process monitoring is becoming increasingly relevant in biotechnology, especially in the manufacturing sector to promote cost-effective production. To date, biotechnical processes are generally monitored using mainly off-line analytical procedures.12 These methods generally involve more or less frequent liquid sampling and are thus problematic in terms of sterility. Considerable time delays between sampling and achieving the analytical results may be encountered. Moreover, these off-line procedures are often labor-intensive and require intensive sample preconcentration or the like. In contrast, PTR-MS overcomes these obstacles, allowing direct, real-time quantification without the need for complex sample preparation.6
In a bioreactor, VOCs are emitted as byproducts of microbial growth and production. With real-time monitoring via the PTR-MS technique of the emitted VOCs in the bioreactor’s headspace, it is possible to gauge the activity of these microorganisms and to gain information for better control of the fermentation process. Of central importance in this context is fast and quantitative transfer of the sample gas into the analytical device. A suitable coupling system that allows the PTR-MS instrument to be connected to a bioreactor in order to monitor fermentor off-gas in real time has been developed.13 This system consists of a specially coated inlet line, a sterility barrier for the bioreactor, and a number of additional safety measures, reducing the likelihood of fermentation broth from accidentally passing through the system to the PTR-MS device. Since samples are taken from the off-gas, the PTR-MS does not interfere with the fermentor’s standard mode of operation, which facilitates the installation with respect to legal regulatory requirements.