Illicit drugs are listed under five categories, namely, narcotics, stimulants, depressants, hallucinogens, and cannabis. These categories include drugs that are produced legally and prescribed by doctors as well as those that are produced illegally and sold outside of medical channels. 1 While each drug of abuse has different physical effects, they all alter the areas of the brain responsible for self-control, judgment, emotional regulation, motivation, memory, and learning.2
The World Drug Report 2009,3 produced by the United Nations Office on Drugs and Crime, presents comprehensive information on the illicit drug market. According to the report, some encouraging reductions in the production of cocaine and heroin were recorded in 2008. Surveys of users in the world's largest markets for cannabis, cocaine, and opiates suggest that these markets are shrinking. In contrast, there are several indications that the global production, trafficking, and use of amphetamine-type stimulants are worsening. In addition, it is estimated that between 11 and 21 million people worldwide inject drugs, with China, the U.S., Brazil, and the Russian Federation having the largest populations of injecting drug users. Together these countries account for 45% of the total estimated worldwide population of injecting drug users.
In order to safeguard human health from the illicit drug threat, stringent legislation is being enforced worldwide. In the U.S., the Drug Enforcement Administration enforces the Controlled Substances Act (CSA),4 which regulates the manufacture, import, export, possession, use, and distribution of psychoactive drugs. The Act includes all drugs that have a stimulant, depressant, or hallucinogenic effect on the central nervous system and as such appear to have abuse potential. The CSA categorizes controlled substances under five schedules according to their medical treatment acceptance, safety for use, potential for abuse, and risk of causing psychological or physical dependence.
The European Union Drugs Action Plan for 2009-20125 includes wide-ranging measures to strengthen European cooperation to curb the adverse consequences of drug use and eliminate drug-related crime. The plan is conceived around five priorities: reducing the demand for drugs, mobilizing European citizens, reducing drug supply, improving international cooperation, and improving understanding of the drugs phenomenon. Additionally, the Council Framework Decision 2004/757/ JHA6 sets minimum provisions on the constituent elements of criminal acts and penalties in the field of illicit drug trafficking.
Under the regulations, severe imprisonment and fine penalties are enforced in cases of unlawful acts. The severity of the penalties depends mainly on the quantity of the controlled substance detected in a drug mixture. As a consequence, a method is required that is capable of separating and detecting illicit drug mixtures in a reliable manner.
Analytical techniques
GC-MS has traditionally been employed for the separation and identification of drugs and metabolites in forensic toxicology, using electron ionization (EI) or chemical ionization (CI).7 This methodology has become a “gold standard” in terms of admissibility and defensibility in court because of its sensitivity, excellent selectivity, and high degree of standardization.8 However, the technique usually involves additional manual derivatization procedures and sample cleanup.
LC-MS methods eliminate the need to derivatize and often simplify sample preparation. Nevertheless, long run times and low separation efficiency limit the utility of conventional HPLC. Ultrahigh-performance liquid chromatography (UHPLC) performs separations 5–10 times faster than conventional HPLC by employing sub-2-µm-diam particles. The 1–2 sec peak widths and relatively high separation efficiency of UHPLC with capillary GC make UHPLC-MS a viable alternative method for illicit drug analysis due to its increased speed and accuracy as well as improved sensitivity An experiment was performed to illustrate the separation and detection of a mixture of 14 illicit drugs/metabolites using UHPLC-MS.
Experimental
Pseudoephedrine, ephedrine, amphetamine , methamphetamine (MA) , 3,4-methylenedioxy-N-methamphetamine (3,4-MDMA), oxycodone, hydrocodone, clonazepam, noscapine, cocaine, caffeine, tetrahydrocannabinol (THC), cannabinol, and cannabidiol standards (1 mg/mL in methanol) were purchased from Alltech-Applied Science (State College, PA). These 14 compounds were mixed with the optimized molar ratio in the range of 1–100 and diluted to 0.1 ppm with methanol to produce the drug mixture standards. Chromatographic analyses were performed using the Thermo Scientific Accela UHPLC system (Thermo Fisher Scientific, San Jose, CA). MS analysis was carried out on the Thermo Scientific MSQ Plus single-quadrupole LC-MS detector.
Results and discussion
MS detection
Both positive and negative electrospray analysis were performed using the polarity switch function of the Windows®-based Thermo Scientific Xcalibur software platform. All of the analytes exhibited higher ionization efficiency in the positive ion mode compared with the negative mode. The MS spectra of the drug standards demonstrated both molecular ion signals of [M+H]+ and acetonitrile (ACN) adducts of the form [M+ACN+H]+. For 13 of the analytes, the signal from the molecular ion was more intense than the signal from the acetonitrile adduct. For amphetamine, the most intense signal was from the acetonitrile adduct [M+ACN+H]+ at m/z of 177.2.
Separations with standard stationary phases
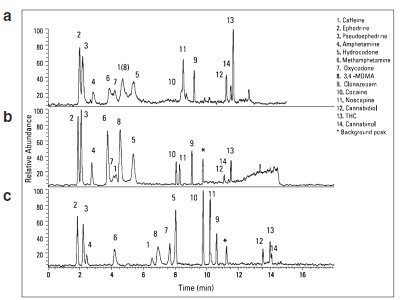
Figure 1 - Comparison of 1.9-µm Hypersil GOLD stationary phases for the UHPLC separation of 14 illicit drugs: a) Hypersil GOLD aQ, b) Hypersil GOLD, c) Hypersil GOLD PFP.
The Thermo Scientific Hypersil GOLD, Hypersil GOLD aQ, and Hypersil GOLD PFP were evaluated to separate the illicit drug mixtures (Figure 1). The UHPLC method with each column type was optimized individually. Hypersil GOLD aQ, a polar endcapped C18 phase that offers more retention of polar compounds, did not resolve the early-eluting compounds, including methamphetamine, oxycodone, caffeine, MDMA, and hydrocodone.7 The separation on the column may have been impaired by interactions between the polar endcapped stationary phase and the polar analytes.
Hypersil GOLD, with LI or C18 selectivity, showed improved selectivity for all analytes except caffeine (peak 1) and oxycodone (peak 7). The column uses a highly pure silica and endcapping procedure to minimize unwanted interactions between analytes and the acidic silanols of the silica support. Hypersil GOLD PFP enabled the optimal separation of all 14 analytes by improving the resolution of the earlier-eluting compounds. The column introduces a fluorine group into the stationary phase to improve selectivity toward halogenated compounds, as well as polar compounds containing hydroxyl, carboxyl, nitro, or other polar groups.9
Separations using acetic acid and trifluoroacetic acid as eluent modifier
Trifluoroacetic acid (TFA), formic acid, and acetic acid can be added to the mobile phase to generate differences in selectivity. Separation of 14 illicit drugs on a Hypersil GOLD PFP column was evaluated by using either TFA, formic acid, or acetic acid as eluent modifier. The separation method with 0.02% TFA (Figure 2a) provided fast separation performance with good resolution and sharp peaks. However, the use of TFA is generally not recommended with MS detection due to its effect on signal suppression.
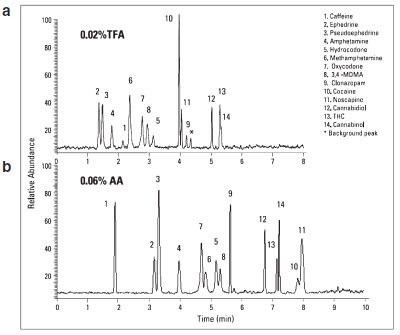
Figure 2 - UHPLC-MS chromatograms of the 14 illicit drugs with acidic solvent modifiers: a) 0.02%, b) 0.06% acetic acid.
All of the analytes were well resolved with 0.1% formic acid as modifier (Figure 1c), but only when 100% water was used at the beginning of the gradient method. However, prolonged use of 100% water may degrade the stationary phase and shorten the column lifetime; thus this gradient method is not suited for routine use. Most of the analytes were well separated with adequate resolution using 0.06% acetic acid as eluent modifier (Figure 2b). However, under such conditions, a few pairs of compounds—such as oxycodone and methamphetamine (peaks 7 and 6), hydrocodone and 3,4-MDMA (peaks 5 and 8), and cocaine and noscapine (peaks 10 and 11)—were not baseline resolved.
Separations with hybrid column phases
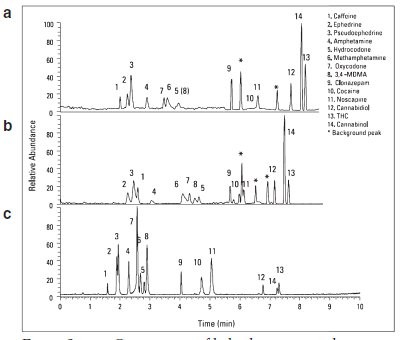
Figure 3 - Comparison of hybrid stationary phase chemistry for the separation of 14 illicit drugs: a) 50 × 2.1 mm Hypersil GOLD + 50 × 2.1 mm Hypersil GOLD PFP, b) 50 × 2.1 mm Hypersil GOLD PFP + 50 × 2.1 mm Hypersil GOLD, c) 100 × 2.1 mm Hypersil GOLD PFP + 20 × 2.1 mm Hypersil GOLD.
Three hybrid stationary phases were evaluated after connecting different stationary phase columns in series (Figure 3). Separations of 14 illicit drugs with these three hybrid stationary phases demonstrated great variation in selectivity. In general, the hybrid column phases improved selectivity between THC and cannabinol, and cocaine and noscapine, but reduced selectivity between earlier-eluting compounds, such as oxycodone, MA, hydrocodone, and MDMA, compared with the Hypersil GOLD PFP phase.
Separation with ternary gradient
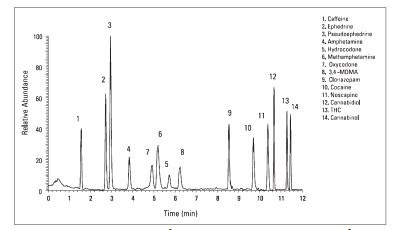
Figure 4 - Optimized UHPLC-MS separation of 14 illicit drugs with ternary gradient.
The separation of the drug mixtures was dramatically improved by using three solvents: water, acetonitrile, and methanol (Figure 4). Baseline resolution of all 14 drugs was achieved. Methanol, a weaker eluent compared with acetonitrile, provided better resolution for most of the analytes. However, the flow rate had to be reduced to accommodate high column backpressure caused by the high viscosity of methanol. Adding acetonitrile reduced the column backpressure so as to maintain the same separation speed.
Calibration curve and sensitivity
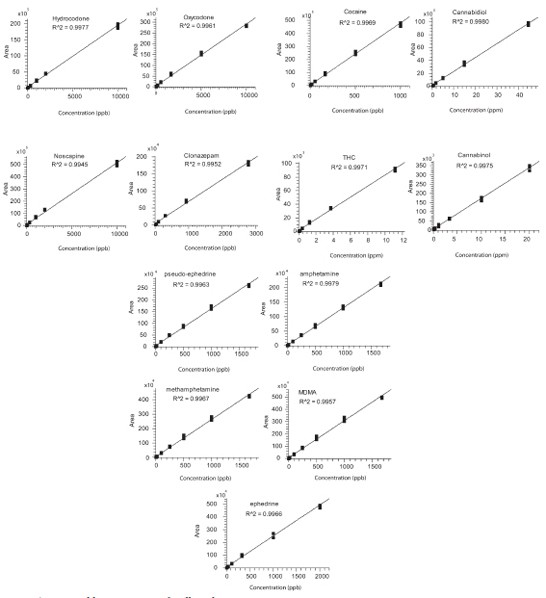
Figure 5 - Calibration curves for illicit drugs.
Calibration curves for the drug standards were constructed over the prespecified concentration range with 10 calibration levels (Figure 5). Each calibration level was injected three times and the mean area responses were plotted against the concentrations. Correlation coefficients with R2 = 0.995 or better were achieved for all illicit drug compounds.
The limit of quantitation (LOQ) and limit of detection (LOD) of the drug compounds were determined based on the calibration curve of signal-to-noise ratio versus concentration and the definitions of LOQ and LOD using S/N = 10 and 3, respectively. LOQs for all drugs were in the range of 0.96–300 ng/mL, while LODs were from 0.29 to 90.0 ng/mL. The outstanding sensitivity of this method was highlighted by the achievement of pictogram-level quantitation for 10 illicit drugs with 1 µL sample injection.
Conclusion
Fast, efficient, and reliable separation and identification of illicit drugs and metabolites are required in order to comply with stringent legislation and protect human health. Using UHPLC-MS with a ternary solvent gradient provides rapid baseline separation of illicit drugs and metabolites. Various selectivities are achieved by employing different column surface chemistries, acidic solvent modifiers, and eluent systems. Following UHPLC separation, detection by single quadrupole MS at the ppb (ng/mL) level is more than sufficient to identify and quantify illicit drugs and metabolites in real samples.
References
- Central Intelligence Agency, Publications. The World Factbook, Illicit Drugs; https://www.cia.gov/library/publications/the-world-factbook/fields/2086.html
- Help Guide. Drug Abuse and Addiction, Signs, Symptoms and Help for Drug Problems and Substance Abuse; http://helpguide. org/mental/drug_substance_abuse_addiction_signs_effects_treatment.htm.
- United Nations Office on Drugs and Crime. World Drug Report 2009 Executive Summary; http://www.unodc.org/documents/ wdr/WDR_2009/Executive_summary_LO-RES.pdf .
- U.S. Drug Enforcement Administration. Title 21-Food and Drugs; Chapter 13- Drug Abuse Prevention and Control, Subchapter I-Control and Enforcement, http://www.justice.gov/dea/pubs/csa.html.
- EU Drugs Action Plan for 2009-2012, (2008/C 326/09); http://www.emcdda.europa.eu/attachements.cfm/att_66226_EN_EU%20drugs%20action%20plan%20for%202009-2012-EN.pdf.
- Council Framework Decision 2004/757/JHA of October 2004 laying down minimum provisions on the constituent elements of criminal acts and penalties in the field of illicit drug trafficking, Official Journal L 335, 11/11/2004; pp. 0008-0011, http://eur-lex.europa.eu/LexUriServ/LexUriServ.do?uri=CELEX:32004F0757:EN:HTML.
- Koeppel, C.; Tenczer, J. Scope and limitations of a general unknown screening by gas chromatography-mass spectrometry in acute poisoning. J. Am. Soc. Mass Spectrom. 1995, 6, 995-1003.
- Weinmann, W.; Wiedemann, A.; Eppinger, B.; Renz, M.; Svoboda, M. Screening for drugs in serum by electrospray ionization/collision induced dissociation and library searching. J. Am. Soc. Mass Spectrom. 1999, 10, 1028-37.
- Chromatography Columns and Consumables, 08-09, Thermo Fisher Scientific; pp 72-82.
Dr. Jiang is Applications Manager, LC/LC-MS Marketing Dept., Thermo Fisher Scientific, 355 River Oaks Pkwy., San Jose, CA 95134, U.S.A.; tel.: 408-433-4800; fax: 408-965- 6118; e-mail: [email protected].