Companies that create personal care products such as cosmetics often perform shelf-life testing to determine stability. Viscosity measurements are an important tool for materials characterization and a sensitive measure of material changes. Many of the formulations are complex emulsions with structures that vary over time. The structural changes may be reflected in the viscosity changes. The viscosity often increases as emulsified structures further develop and equilibrate. In other cases, over long storage times, the viscosity may significantly decrease as product degradation occurs. This article focuses on a rotational viscometer (or rheometer) as the measuring instrument. A spindle is immersed and rotates in the sample. As the liquid resists the rotation, the torque is measured by a spring that winds up while rotating inside the instrument. A digital viscometer has an electronic sensing system that reads the spring deflection. The on-board firmware then converts the torque reading into viscosity, accounting for the instrument’s spring torque range, spindle, and speed (in rpm). Data are considered to be on-scale for torques 10–100% of full-scale range (FSR). FSR corresponds to 100%. Figure 1 shows a typical, programmable, digital viscometer.
There is concern that a useful QA/QC test, performed at one point in shelf-life, with a given torque range, spindle, and speed, may no longer provide on-scale data at a significantly different time, months later. One way to handle this is to use test conditions such that the measurement is at 50% of full-scale range, at given conditions of range, spindle, and speed. For the purposes of this discussion, the same container was used for testing throughout the shelf-life of the product. Setting the initial test conditions to achieve approximately 50% of full scale may allow enough leeway to provide subsequent on-scale measurements, whether the viscosity increases or decreases.
Some viscosity changes may be great enough so that the speed and/or spindle may have to be changed, although the same torque range may be used. One standard operating procedure (SOP) may be created for the initial test of freshly prepared material, and another SOP for the viscosity test of material aged X number of months. Another approach for testing materials undergoing very large viscosity changes is to use instruments with two different torque ranges, but having identical or very similar speeds with the same spindle.
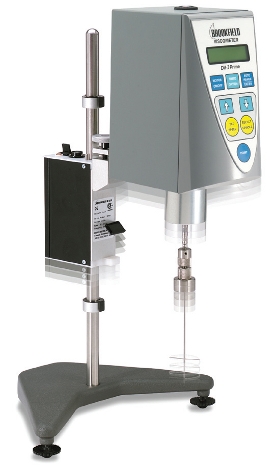
Figure 2 – Helipath Stand with viscometer.
These materials are often non-Newtonian, that is, the measured apparent viscosity is a function of shear rate. Shear rate in turn depends upon 1) the geometry, including the spindle and sample container shape and size; and 2) the test (rotational) speed. Therefore, consistency of method is key. The same exact experimental conditions should be used each time a given material is tested.
Geometries for materials that may yield or slip
Numerous personal care products, particularly creams and very viscous lotions, have yield stresses—a form of non-Newtonian behavior. The yield stress is the stress at which a solid-like material begins to flow as a liquid. Furthermore, a significant proportion of these products exhibit either total structural breakdown within the yielded region and/or slip. Slip is essentially a significant or complete lack of “tack” or “grip” between the sample material and the spindle. Petroleum jelly, also known as petrolatum, is a classic example: The material fractures and the spindle essentially “drills a hole” into the sample. Certain cold creams appear to be soft solids, and then flow easily when pressed with the user’s fingers. The deformed regions of the petroleum jelly and cold creams do not appear to rebuild their structures after the shearing stops.
Smooth geometries such as cone-plate, coaxial/concentric cylinder, or parallel plate may not provide valid data in these cases. Therefore, many operators use the Helipath™ Stand Model D system (Brookfield Engineering Laboratories, Inc.), in which a motorized stand lowers a viscometer head while a thin T-shaped spindle rotates at a low to moderate speed, such as 10 rpm or less (see Figure 2). Figure 3 shows typical Helipath data for a petroleum jelly.
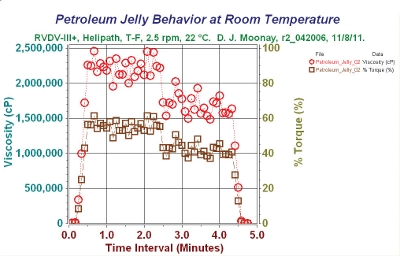
Figure 3 – Typical Helipath data for a petroleum jelly.
The spindle effectively carves a helical path down through the sample, continuously testing fresh material. Several data points are taken over time, and the results are averaged to provide a meaningful QA/QC measure. As shown in Figure 3, the torque and calculated viscosity increase as the spindle proceeds down into the sample, and the reading plateaus in the bulk of the sample. The data from this plateau are suitable for averaging.
The Helipath drive unit then reverses direction. The resulting data values, from about 2.5 min onward, are somewhat lower than before, because the spindle proceeded through material that was partially disturbed in the previous portion of the test. The readings finally approach zero as the spindle rises up out of the sample.
This technique provides very useful correlations to process engineering data for certain rheologically complex products. However, there are no defined shear rates. Operators should use procedures that are convenient and that give them meaningful, reproducible data. The Helipath and T-bar spindles are suitable if all they need is a reproducible viscosity number without shear rate.
Vane spindles (Figure 4) are another geometry useful for products that slip and exhibit a yield stress. Vane spindles are helpful for measuring both viscosity and yield stress. Figure 5 presents petrolatum viscosity data obtained with a vane spindle. The graph strongly suggests yield behavior. The measured torque and calculated apparent viscosity first rise, during testing at a constant rotational speed, over time, and then peak and sharply decline.
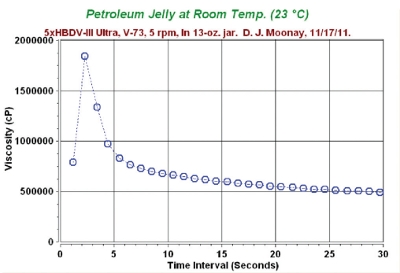
Figure 5 – Typical vane data for a petrolatum product.
Vane rheometry is also useful in measuring yield stress—the stress required to make a solid-like material flow like a liquid. Yield stress correlates with suspension stability over time. As structure builds up and strengthens, the yield stress increases. Structural breakdown and weakening are often well-correlated with a decreasing yield stress. Figure 6 shows yield stress data for a petroleum jelly.
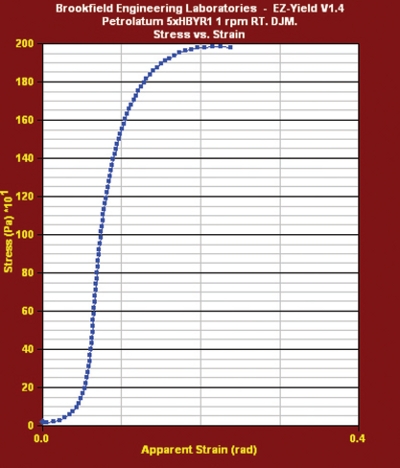
Figure 6 – Typical yield stress data for a petrolatum product.
The measured stress increases as the vane rotates. The maximum stress value in Figure 6 is the yield stress. The test automatically stops when a significant, user-specified, decrease from the peak value is detected. The apparent strain is the amount that the sample is deformed during the test.
Choosing the right method for viscosity measurements
Users sometimes wonder which method may be preferable, when certain combinations of spindles and speeds cover overlapping viscosity ranges, with a given spring torque range. In one case, on-scale data were taken using a smaller spindle at 5 rpm and with a larger spindle at 2.5 rpm. The user traditionally tested product “A” using the larger spindle at 2.5 rpm. However, that particular lotion seemed similar in use to other products that were tested with a smaller spindle at 5 rpm. The operators wondered whether it would be advantageous to change product A’s test to match that of the other materials.
The answer depends on the priority of the user. If data may be acquired faster at 5 rpm, because the instrument’s spring winds up and mechanically equilibrates faster at 5 than at 2 rpm, and every minute counts, then it may be worthwhile to change to the more rapid test. However, if years of historical data taken with the original test must be matched, and the material is non-Newtonian, then it may be imperative to follow the existing method. One compromise may be to run parallel tests of the same material with both methods, and develop an experimental correlation, in order to transition to the new method.
Conclusion
Rotational viscometry/rheometry is a useful method for analyzing shelf-life. Appropriate selection of spring torque ranges, spindles, and speeds may be used to monitor viscosity (or yield stress) changes over time with a consistent technique.
David J. Moonay, Ph.D., is Sales Engineer—Rheology Laboratory Supervisor, Brookfield Engineering Laboratories, Inc., 11 Commerce Blvd., Middleboro, MA 02346, U.S.A.; tel.: 508-946-6200, ext. 7144; e-mail: [email protected] ; www.brookfieldengineering.com.