The invention of atomic force microscopy (AFM)1 has revolutionized contemporary microscopy and empowered researchers with a unique method to study materials at the submicron scale. Recently, the strong interest in nanoscale science and nanotechnology further promoted AFM and its applications. The ability to examine samples in different environments/temperatures, and obtain structural and compositional information at scales down to atomic dimensions makes this technique an invaluable companion to traditional optical and electron microscopy. The development of commercial atomic force microscopes brought AFM into research laboratories and manufacturing facilities in a number of industries. At present, over a dozen companies are making scanning probe microscopes, and there are also several manufacturers of AFM probes and suppliers of accessories. This makes it difficult for a newcomer to choose a microscope and probes that will best suit his or her research needs. Currently, the quality of microscopes is similar, and subjective factors often prevail in making a choice.
In past years, progress in AFM instrumentation has slowed down. Advances were made rather by adding accessories and introducing low-cost microscopes than by developing core microscopic functions such as high-resolution imaging and studies in different environments. The situation is different in research communities in which scientists are continuously exploring new ways of improving image resolution, imaging at different environments, and conducting novel mechanical and electromagnetic measurements at the submicron scale. This work will inevitably impact AFM instrumentation. Several AFM applications that require further development will be described based on the author’s experience with the Agilent 5500 microscope (Agilent Technologies, Inc., Chandler, AZ).
Imaging with atomic and molecular resolution was the key feature that brought many researchers to the field of scanning tunneling microscopy (STM) and AFM. STM images of semiconductors, metals, and organic conductors exhibit periodical patterns that closely resemble crystalline lattices of these compounds. In addition, one can also observe single atomic-size defects such as vacancies that are considered proof of true atomic resolution. In AFM, visualization on the atomic scale is a complicated phenomenon. In the contact mode, atomic-scale images of crystals reveal their lattice order, but single defects are not observed.2 Therefore, the value of these observations is limited.
The introduction of oscillatory AFM techniques, such as amplitude modulation (AM) and frequency modulation (FM) modes, enabled further expansion of AFM applications. In these modes, destructive lateral forces common to the contact mode are practically eliminated, and AFM applications were expanded to a broad range of soft biological samples and polymers. Atomic- and molecular-scale imaging in oscillatory techniques was first demonstrated in the FM of semiconductor surfaces in ultrahigh vacuum (UHV).3,4 In contrast to the contact mode, researchers have detected single atomic-scale defects and claimed true atomic resolution in FM. The combination of FM and UHV was considered necessary for atomic-scale imaging until atomic resolution was achieved in the FM of several compounds in air and water.5,6 The latter results were obtained with improved instrumentation in the university laboratory.
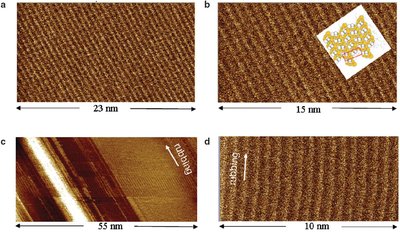
Figure 1 - Molecular-resolution AFM images obtained on the crystallographic bc plane of PDA crystal (a, b) and on oriented PTFE layer. The insert in (b) shows a molecular arrangement on the PDA crystal simulated from the X-ray diffraction study. White arrows in (c) and (d) indicate the direction of hot rubbing on a glass substrate during preparation of the PTFE sample.
Simultaneously, the true molecular resolution was achieved in AM mode on polydiacetylene (PDA) crystal in air using a commercial microscope.7 This finding is supported by the results obtained with the Agilent 5500 microscope in air on PDA crystal and an oriented polytetrafluoroethylene (PTFE) layer. The molecular-scale images of PDA (Figure 1a and b) correspond well to the molecular arrangement at the bc crystallographic plane (b = 0.491 nm; c = 1.410 nm). The high-magnification image in Figure 1b shows that the neighboring molecular rows aligned along the b axis are not identical. This may be a result of a slight surface reconstruction that takes place when a fresh surface of the crystal is formed by a cleavage. The images of the PTFE sample (Figure 1c and d) show surface regions formed by layers of oriented single macromolecules, which are distinguished as bright strips aligned along the rubbing direction. The spacing between the neighboring strips is ~0.56 nm and it corresponds to the interchain distance found in the X-ray studies of highly oriented PTFE. Despite these findings, the interpretation of the high-resolution images might be rather difficult. Recent modeling studies8 revealed that an observation of single molecular defects does not necessarily prove that a nearby lattice pattern reflects a real molecular arrangement. The image varies drastically when imaging is performed at elevated forces with tips of a few nanometers in diameter. True atomic resolution can be achieved when ultrasharp probes are used for imaging at very low forces and at the conditions of low thermal drift.