pH influences the rate of many chemical and enzymatic reactions. Therefore, buffered solutions are commonly utilized in the laboratory. These solutions are usually prepared with high-purity water in order to minimize the risk of contamination with water impurities. This paper not only describes the difficulties and alternatives to measuring the pH of high-purity water, but also the impact of buffer dilution on pH.
pH of high-purity water
Table 1 - A quick reference guide of basic definitions and equations
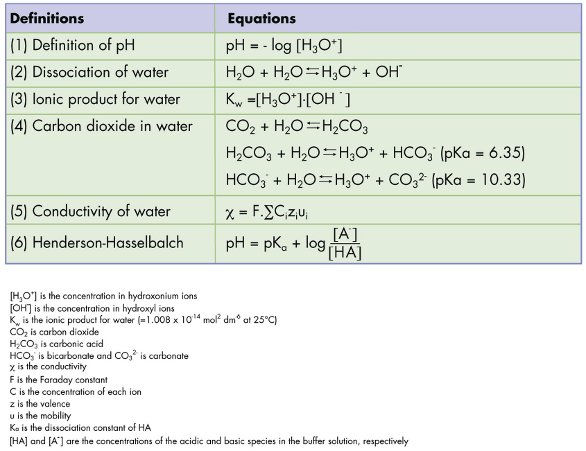
The pH of water is defined as the negative logarithm of the hydrogen ion activity, which is often assimilated to the hydrogen ion concentration (Eq. [1], Table 1). While the pH of pure water is approximated to be 7.0 at 25 °C, the theoretical value is 6.998. Indeed, the dissociation of water and the ionic product for water (Eqs. [2] and [3], Table 1)1 indicate that 10–6.998 mol/L of H3O+ are present in pure water. However, one cannot measure the pH of high-purity water simply by dipping the electrodes of a pH meter into a beaker full of water. This measurement is challenging due to the quasi-absence of ions that would enable electron transport between the measuring and reference sides of the pH electrode. It often results in erratic and meaningless pH readings.
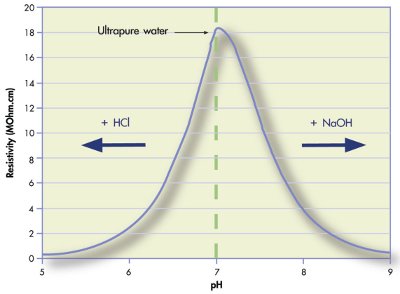
Figure 1 - Theoretical resistivity values of water following the addition of hydrochloric acid (HCl) or sodium hydroxide (NaOH). The resistivity of ultrapure water is 18.18 MΩ.cm and the pH is 6.998.
Adding the slightest amount of acid or base to pure water will change its pH significantly (Figure 1). Pure water readily absorbs carbon dioxide (CO2) when exposed to the atmosphere, which forms carbonic acid (Eq. [4], Table 1). Carbonic acid dissociates into bicarbonate that is in equilibrium with carbonate. Dissolution of CO2 in water ultimately leads to a pH of approximately 5.8. It is this pH value that is eventually obtained if one measures the pH of ultrapure water without working under controlled conditions.
The issues linked to the pH measurement of ultrapure water are addressed in various references. The United States Pharmacopeia (USP) recommends the addition of potassium chloride (KCl) to alleviate the problem.2 A more precise and complex experimental setup and method for the pH measurement of pure water are described in an American Society for Testing and Materials (ASTM) Standard Test Method.3 It includes the use of a flow-through sample chamber and the addition of KCl via a reference electrode with positive electrolyte leakage. Other standards, such as the International Organization for Standardization (ISO) 3696 standard, simply do not require the pH measurement of pure or ultrapure water. They rely on other physicochemical parameters to characterize water quality, such as conductivity.
A conductivity measurement may be used as an alternative to the pH measurement of high-purity water. Conductivity measures the flow of electrons through a fluid, which is proportional to the concentration of ions, their charge, and mobility (Eq. [5], Table 1). The relationship between resistivity (the reciprocal of conductivity) and the pH of water is illustrated in Figure 1. When only pure water is present, a conductivity value of 0.055 S.cm–1 at 25 °C is measured. This results from the water dissociation into hydroxide and hydroxonium ions. Therefore, at 25 °C, a conductivity of 0.055 S.cm–1, or a resistivity of 18.18 MΩ.cm, implies that the water is ultrapure and that the pH is inherently 6.998.
Diluting buffer solutions
The pH of a buffer solution composed of a weak acid and its conjugate base is related to the dissociation constant (pKa) of the acid/base pair, as shown in Eq. [6], Table 1. By definition, buffers are resistant to large changes in pH. However, in the laboratory setting it becomes evident that diluting a buffer with pure water impacts the pH of the solution. Several parameters might explain these pH variations.
- Temperature. Activity coefficients and equilibrium constants vary with temperature, which causes the pKa and pH of a buffer to change. Temperature coefficients vary significantly with the nature of the buffer (–0.028 pH/°C for Tris and –0.0028 pH/°C for phosphate). For this reason, temperature is usually reported along with pH values. The pH of water itself varies with temperature: 7.27 at 10 °C, 7.00 at 25 °C, and 6.63 at 50 °C.
- Buffer capacity. Buffer capacity is the ability of a buffer to resist changes in pH. It depends on the total concentration of the acid/base pair and the concentration ratio. Buffer capacity is high when solutions are concentrated, the ratio of acid to base is close to 1, and the pH is ±1 unit from the pKa.
- Ionic strength. Ionic strength describes the amount of electric charges in a solution. It is a function of the concentration and the valence of the ions present. High ionic concentration tends to limit the mobility of the hydrogen ion, which decreases its activity and influences the pH measurement.
- Dilution. Related to a decrease in ionic strength, dilution directly impacts the pH of a solution. For example, the dilution of a 0.1 M buffer system (HA/HA–) with an equal volume of water results in a pH change of 0.024 units.4 The pH variation of HA–/A2– buffer systems, such as phosphate, is three times that value.
Buffers are often prepared by diluting stock solutions. In this study, two phosphate buffers were diluted 10 and 100 times with various water qualities and pH was measured. The two buffer solutions were a phosphate buffer (1.0 mol/L, pH 7.4) and a phosphate buffered saline 10× concentrate (PBS, 0.1 mol/L, pH expected to be 7.2–7.6 after a tenfold dilution), both from Sigma-Aldrich (St. Louis, MO). Three types of water were used: 1) pure water delivered by an Elix® 10 UV water purification system (Millipore Corp., Billerica, MA), 2) ultrapure water produced by a Milli-Q® (Millipore Corp.) Biocel® (Velocity11, Menlo Park, CA) purification system, and 3) bottled Gibco™ distilled water (Invitrogen, Carlsbad, CA). Experiments were performed in triplicate.
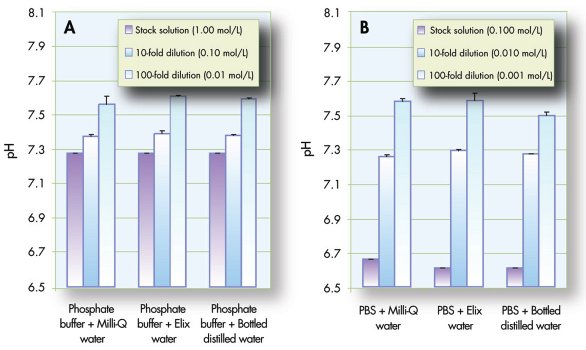
Figure 2 - Effect of phosphate buffer dilution with high-purity water. Values shown are the mean and standard deviation of three samples: a) phosphate buffer (1.0 mol/L, pH 7.4), b) 10× phosphate buffered saline (PBS, 0.1 mol/L).
Diluting phosphate buffers with water resulted in pH increases (Figure 2). For each buffer, the pH changes were similar with all three types of water. The tenfold dilution of the 10× PBS solution resulted in a pH close to the expected value of 7.4. The PBS solution had a lower buffer capacity than the phosphate buffer (lower concentration—0.1 M for PBS versus 1.0 M for phosphate—and original pH farther from pKa) as well as higher ionic strength. This explains the larger change in pH upon initial dilution of PBS compared to phosphate buffer: 6.7–7.3 versus 7.3–7.4.
Conclusion
High-purity water is often used in the laboratory to avoid possible artifacts caused by water impurities. Measuring the pH of this water is challenging and necessitates specialized equipment. However, using water with high resistivity (18.2 MΩ.cm at 25 °C) ensures that the pH is close to 7.0. Diluting buffer stock solutions with high-purity water leads to pH changes. These variations in pH are unavoidable and can be explained by changes in ionic strength and buffer capacity.
References
- Nora, C.; Mabic, S.; Darbouret, D. Ultrapure Water2002, 56–60.
- United States Pharmacopeia National Formulary, USP 23 NF18, 1995, 1637.
- Standard Test Methods for pH Measurement of Water of Low Conductivity. ASTM D 5464–93 (reapproved 1997).
- Perrin, D.D.; Dempsey, B. Buffers for pH and Metal Ion Control; John Wiley & Sons: New York, 1974.
The authors are with Research and Development, Bioscience Division, Millipore Corp., Boîte Postale 307, F-78094 St. Quentin en Yvelines, France; tel.: +33 1 30 12 71 40; fax: +33 1 3 12 71 11; e-mail: [email protected].