The physicist Richard Feynman famously said in reference to (what is now called) nanotechnology, that “there is plenty of room at the bottom.”1 While the point of this comment and the thrust of his speech were aimed at the concept of synthetic nanomachines, he began by noting how effective biological systems are in their economy of material usage. The goal, then, for the biologist is to understand the organization and function of these nanomachines. In the same presentation, Feynman provided advice for the biologist: “Just look at the thing!” He then volunteered the hypothetical response of the biologists to the physicists: “What you should do in order for us to make more rapid progress is to make the electron microscope 100 times better.” Advanced scanning electron microscopy (SEM) technology is able to support this progress.
A new technology—the helium ion microscope (HIM)—provides subnanometer-resolution imaging with high surface sensitivity that may indeed open up the door to further rapid advancement. This article describes a specific imaging project recently undertaken by the authors. The aim is not so much to answer the research question (about collagen fibers, in this case), but to present an example of a situation encountered several times by the authors in their exploration of biological imaging. There is plenty to see at the bottom.
Project background
Today’s SEM tools are built on established technologies and have many well-developed capabilities. These have much to offer in biological sample imaging. The business unit within Carl Zeiss SMT (Peabody, MA) has developed a charged particle microscope that relies on an ion source that produces an extremely bright beam of helium ions. The technology has been described previously.2,3 The microscope performance is characterized most significantly by high resolution, surface sensitivity, and the ability to image insulating samples without conductive coatings, since charge neutralization is provided by an integrated, low-energy electron flood beam. The high brightness of the source allows a focused probe diameter of 0.35 nm to be obtained in the current tool.
The imaging is characterized by high surface sensitivity. Two factors contribute to this: 1) The impinging helium ions cannot transfer much energy to electrons in the sample due to the large ion-electron mass mismatch. Therefore, only electrons generated within a few nanometers of the sample surface are able to escape from the sample and contribute to the image. 2) There are no backscattered primary electrons as in a scanning electron microscope. In SEM, these scatter from deeper within the sample, and during their escape through the surface they generate secondary electrons that are also detected, but these are not local to the probe landing position. As the SEM beam energy goes up, this nonlocal signal mutes more and more of the true surface information. Thus, the HIM surface information is equivalent to that of an SEM operated at a couple of hundred volts (which is difficult to achieve), while the resolution performance is beyond even that of a high-energy SEM. Both surface information and resolution are achieved simultaneously. Finally, for some materials (e.g., collagen) there is no observed alteration of the sample structure, even after high ion doses. The combination of the two microscopy techniques can be applied to reveal significant structural information of those truly nanomachine-sized objects from 1 to 10 nm in size, and therefore offers a good opportunity to “just look at the thing” and learn much more.
Experimental
One of the sample classes being investigated is collagen. There are over two dozen forms of collagen, and it is present in a wide variety of human tissues. Defects in collagen formation are implicated in many diseases. During previous work the authors were able to image a small number of collagen samples, which provided a baseline. These two factors make collagen a strong candidate for a case study.
The work was conducted with Dr. Nigel Fullwood of Lancaster University (U.K.) on an investigation of corneal and sclera tissue collagen. Several samples of fixed, critical point dried, bovine tissue were studied that had not been stained or given a conductive coating. Samples were provided on tacky carbon-surfaced SEM stubs. The goal was to obtain high-magnification imaging of the proteoglycan bridges that support the organization of the collagen fibers.4 The inherent three-dimensional structure of these arrangements cannot be captured easily in transmission electron microscopy (TEM) (tomography is required). For all samples, the collagen was only exposed by pulling the tissue apart and examining the interior; this crude method gave some disturbance to the layout of the fibers.
HIM imaging was done in an ORION® microscope (Carl Zeiss); typical operating beam conditions were 35 keV energy at 0.2–1.0 pA current. The built-in charge neutralization system was used for almost all the imaging, although one area exhibited no charging. SEM work was done with a Carl Zeiss Ultra Plus® microscope operating between 1 and 10 keV. The tool’s Gemini® column allows for very easy beam energy adjustment over the entire range of interest. All of this makes it possible to combine sufficient data from both tools, taking advantage of the resolution and surface sensitivity offerings of each technology, in attempts to capture the three-dimensional relationships between the collagen and the proteoglycans.
Findings
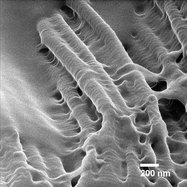
Figure 1 - HIM image of corneal region collagen fibers, 57 kX.
Figure 1 is an HIM image of corneal collagen fibers aligned parallel to one another. The fibers are embedded in a matrix of perpendicular cross-bridges that might be proteoglycans. The D-banding in the fibers can be seen. This is obscured in some areas, however, due to some overlying material. The fibers seem to be totally covered by this coating along the left of the image. The technique’s high surface sensitivity enables even small amounts of surface material to be captured. HIM provided a good 3-D view of the structure.
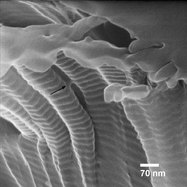
Figure 2 - HIM image of scleral region collagen fibers, 163 kX.
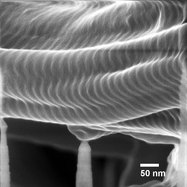
Figure 3 - HIM image of corneal limbus region collagen fibers, 229 kX.
Figure 2 shows a higher-magnification image of the sclera sample. The tissue surface is shown in the upper right-hand corner, with the collagen bundle oriented roughly normal to that surface. The D-banding in the fibers is very distinct, highlighting both the surface sensitivity of the imaging and revealing that there is no surface coating on the fibers, as was observed in the location shown in Figure 1. Since the fibers are not being viewed perpendicular to their length, the D-banding distance appears shorter than the known 65-nm value. The arrow in the figure also points to a bridge structure about 12 nm wide between two fibers. Finally, even with the charging, image resolution of 1.1 nm is obtained.
Figure 3 shows an even higher-magnification image (229 kX) of tissue from the interface of the cornea and sclera. This demonstrates very good surface information as well as a measured image resolution of 0.86 nm. Fine nanometer-scale roughness can be observed on the fibers in the foreground. No charge neutralization was needed in this location, possibly due to the presence of a nearby grounded surface. Thus, HIM is capable of providing nanoscale structural information with a high detail of surface information as well.