Coal-fired utilities have long carried the stigma of being the largest contributor of anthropogenic mercury emissions, which, in 1999, caused the U.S. EPA to initiate the Information Collection Request (ICR). The findings of the ICR indicated that U.S.-based coal-fired utilities emitted between 45 and 48 tons of mercury per year and thus posed a significant threat to the environment. This prompted the U.S. EPA, in March 2005, to issue a rule to cap and reduce mercury emissions from coal-fired utilities, making the U.S. the first country in the world to regulate mercury emissions from such facilities.
At this point in time, the industry had already spent a significant amount of money on the installation of systems to reduce nitrogen oxide and sulfur dioxide emissions. These systems, coincidentally, were also found to reduce mercury emissions. This idea of “co-benefit” prompted the U.S. EPA to take mercury off the list of harmful pollutants. It has become apparent, however, that by simply utilizing co-benefit, the proposed goal of 15 tons of mercury per year, to be met by 2018, will not be achieved. A February 2008 federal courts decision has demanded that mercury be placed back on the list of harmful pollutants that coal-fired utilities have to test for. In March, the Administration asked the U.S. Court of Appeals for the District of Columbia to review and overturn its initial decision. Legal analysis prepared by the Congressional Research Service, however, predicts that the Administration is unlikely to succeed in getting the decision overturned. Additionally, many states, unhappy with the U.S. EPA’s initial actions, have created their own, stricter rules. Thus, with regulations all but looming, it will become critical for coal-fired utilities to have an analytical tool for assessing mercury at their facilities.
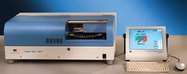
Figure 1 - DMA-80 direct mercury analyzer.
A U.S. EPA draft method, Method 30 B, has been approved as a reference method for relative accuracy testing audits of Continuous Emissions Monitoring Systems (CEMS) and sorbent trap monitoring systems installed at coal-fired utilities. It is also appropriate for mercury emissions testing at such facilities. This method is a procedure for measuring total vapor phase mercury emissions from coal-fired combustion sources using an extractive or thermal analytical technique and UV atomic absorption (AA) or UV atomic fluorescence (AF) cold vapor analyzers as the analytical system. The DMA-80 direct mercury analyzer (Milestone Inc., Shelton, CT) (Figure 1) uses thermal decomposition and ultimately AA spectrophotometry to analyze samples. The analyzer can be used for Method 30 B analysis.
Direct mercury analysis offers the operator numerous benefits. Not only does the elimination of sample preparation save operator time, but also substantially reduces the amount of chemical waste associated with traditional mercury analysis. In addition, low analyte recoveries, caused by either incomplete digestion or the element’s volatility, are negated, ensuring quality data. The DMA-80 typically produces an answer approximately 5 min after a sample has been entered into the decomposition chamber, and has a dynamic range of 0.005–1000 ng per sample boat.
System design
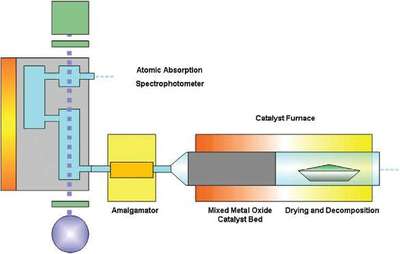
Figure 2 - Diagram showing the entire process within a direct mercury analyzer.
In a direct mercury analysis system, controlled heating is used to first dry and then thermally decompose the sample introduced into the quartz decomposition chamber (Figure 2). A steady flow of oxygen then carries the decomposition products through a catalytic section where halogens, nitrogen, and sulfur oxides are trapped. All mercury species are reduced to Hg(0) and carried through to a gold amalgamator where mercury is selectively trapped. A purging step commences that flushes all nonmercury vapors and decomposition products out of the system. After the purging step, the amalgamator is rapidly heated, releasing mercury vapors to the single-beam, fixed-wavelength atomic absorption spectrophotometer. Here, absorbance is measured at 253.7 nm as a function of mercury content.
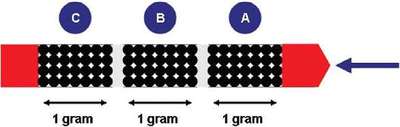
Figure 3 - Three-section sorbent trap.
Table 1 - Sample processing parameters

Table 2 - Experimental results
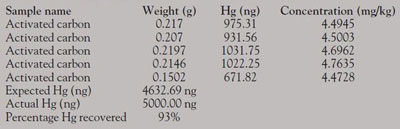
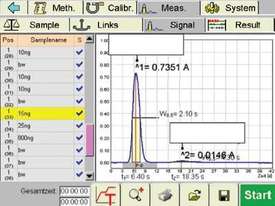
Figure 4 - Typical DMA-80 analysis graph.
Experimental
Sorbent traps typically consist of three sections, each containing approximately 1 g of iodinated charcoal. As emissions pass through the trap, the first section (A) is intended to capture the majority of the mercury, with the second (B) and third (C) sections there to capture any “breakthrough” mercury (Figure 3). For this study, a sorbent trap was spiked by a third party with 5000 ng of total mercury. The sorbent trap was broken and each section was collected individually and homogenized. The individual sections were run, in approximately 200-mg increments, using the program parameters shown in Table 1.
Results
A summary of the results obtained is shown in Table 2. A typical DMA-80 analysis graph is displayed in Figure 4.
Conclusion
Table 3 - Results comparison: DMA-80 versus certified values

The DMA-80 direct mercury analyzer was able to successfully process the iodinated charcoal samples, recovering approximately 93% of the original mercury spike. The total analysis time per sample was less than 10 min, including the time taken to weigh and place each sample into the sample boat. The DMA-80 results obtained when processing coal and coal fly ash Standard Reference Materials (SRMs) are in good agreement with the certified values of those materials (Table 3). Periodically running selected SRMs in sequence with the unknown samples ensures that QA-QC requirements are met and demonstrates the high reliability of the DMA-80.
Mr. Nortje is Mercury Product Specialist, Milestone Inc., 25 Controls Dr., Shelton, CT 06484, U.S.A.; tel.: 203-925-4240; fax: 203-925-4241; e-mail: [email protected].