Antimicrobial materials are widely used in industry, community, and private settings to prevent microbial infection. 1,2 To obtain biocidal effect without releasing biocides into the environment, antimicrobial species can be covalently coupled to the material. Previously, the authors used a natural product, konjac glucomannan (KGM), as a material and quaternary ammonium as an antimicrobial species to covalently synthesize a new chemical, KGM-g-DMAE-BC (N,N-dimethylaminoethyl methacrylate), which was proven to have antibacterial effects.3 However, its mechanism and bactericidal characteristic are still unknown. The small-molecule quaternary ammonium salts exert their antibacterial action by disrupting and disintegrating cell membranes. The authors hypothesized that the active group of KGM-g-DMAE-BC is a quaternary ammonium and designed several experiments to prove it. They first tested its bacterial adsorption kinetics and found that the bacteria were absorbed within 5 min. Next they employed a triphenyltetrazolium chloride (TTC) test to show that the bacteria absorbed on the surface of KGM-g-DMAE-BC were killed. Finally, a DNA and RNA release study was conducted to investigate whether the integrity of the cell membrane was involved in the mechanism of antibacterial effects of KGM-g-DMAE-BC. The results clearly demonstrate the authors’ hypothesis.
Materials and methods
Materials
The synthesis and characterization of konjac glucomannan modified with KGM-g-DMAE-BC used in this study have been described elsewhere.3Escherichia coli 8099 were kindly provided by Dr. Kaijuan Wang, School of Public Health, Zhengzhou University (Henan, China).
Adsorption kinetic study
The quantitative suspension experiments were performed as follows. Briefly, KGM or KGM-g-DMAE-BC (percentage grafting [PG] 38.5% [see Ref. 3]) was mixed with 5 mL 108 colony forming units (CFU)/mL Escherichia coli, respectively. All mixtures were shaken at 37 °C for 5, 10, 15, 20, 25, 30, 40, 50, or 60 min and then kept stationary for 30 min until the supernatant separated from the deposition. One milliliter supernatant of each of the mixtures was diluted 10–106 times, and 0.1 mL diluted solution was incubated at 37 °C for 24 hr to count CFU. The average values of the three duplicates were converted into CFU/mL in the test tube by multiplying the dilution factor.
Testing the integrity of the cell membrane
If the bacteria membrane is compromised, the release of cytoplasmic constituents of the cell such as DNA and RNA can be monitored. One can estimate the amount of DNA and RNA released from the cytoplasm by the detection of absorbance at 260 nm. The experiments were conducted as follows. The bacterial suspension was separated into several flasks. Different doses of KGM-g-DMAE-BC (0.5, 1.0, or 1.5 g) were added to each flask except the control. Samples of 1.5 mL were removed from the flasks every 20 min. The samples were then immediately filtered with 0.2-mm syringe filters to remove the bacteria. The supernatant was then diluted appropriately, and optical density at 260 nm was recorded.
TTC test
Activity of D Hase (dehydrogenase) can be a direct indicator of microbial activity. One of the most frequently used methods is based on the use of TTC as an artificial electron acceptor for several dehydrogenases. The TTC is water-soluble, and nearly all microorganisms reduce it to triphenylformazan (TF), which can be estimated colorimetrically.4

The liquid sample (L) experiments were conducted as follows. A mixture of 90 mL normal saline, 0.5 g KGM-g-DMAE-BC, and 10 mL E. coli (109 CFU/mL) was added to one flask. The control flask used the same mixture except for 0.5 g KGM instead of 0.5 g KGM-g-DMAEBC. Both the control and the experiment were processed in exactly the same way. The mixture was shaken at 37 °C for 30 min, and was then kept stationary for 30 min to separate the supernatant liquid from the solid phase. Two milliliters supernatant liquid was added to a 150-mL flask. Two milliliters glucose solution (1 mol/L) and 2 mL TTC solution (1.0000 g/L) were added to the flask and allowed to react for 30 min. Two drops of H2SO4 were added to terminate the reaction. The hydrolysis reaction product (TF) was extracted for 2 hr at 30 °C with toluene. The TF concentration was then determined spectrophotometrically at 492 nm.5
The solid sample (S) experiments were conducted as follows. The solid of KGM (S) and KGM-g-DMAE-BC (S) was filtered and dissolved in 2 mL normal saline. It was then treated with the supernatant.
Results and discussion
Adsorption kinetic study
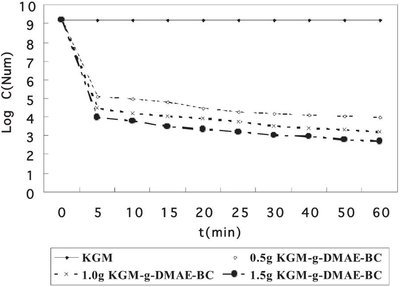
Figure 1 - Adsorption kinetic curve of KGM-g-DMAE-BC and KGM.
Figure 1 shows the adsorption kinetic curve of KGM-g-DMAE-BC and KGM. As can be seen from the figure, 0.5 g KGM did not show any adsorption during the 60 min. In contrast, KGM-g-DMAE-BC (0.5, 1.0, and 1.5 g) dose-dependently absorbed E. coli. During the first 5 min, KGM-g-DMAE-BC drastically decreased the number of bacteria from 109 to 105. However, further incubation with KGM-g-DMAE-BC decreased the number of bacteria, but not as drastically as during the first 5 min. This phenomenon is very interesting, implying that bacterial adsorption of KGM-g-DMAE-BC is fast and can be saturated.
Testing the integrity of the cell membrane
The bacterial membrane serves as a structural component that may become compromised during a biocide challenge such as exposure to a cationic biocide. Therefore, the release of intracellular components such as large molecules, DNA, RNA, and other materials is a good indicator of membrane integrity. Since these nucleotides have strong UV absorption at 260 nm, they are described as 260-nm absorbing materials.6 Using a UV-VIS spectrophotometer, it is very easy to detect any 260-nm absorbing materials; this method is widely used in determining membrane integrity.
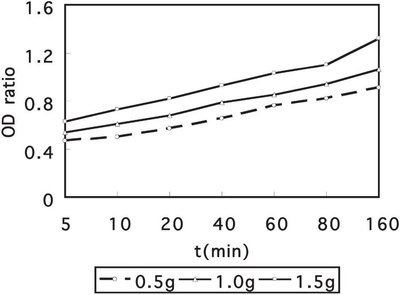
Figure 2 - Release of 260-nm absorbing material from E. coli with addition of 0.5, 1.0, and 1.5 g of KGM-g-DMAE-BC after exposure for different periods of time.
The UV-VIS study on the release of 260-nm absorbing materials upon addition of 0.5 g, 1.0 g, and 1.5 g KGM-g- DMAE-BC to an E. coli suspension is shown in Figure 2. The ratio of O.D. of a bacterial suspension with KGM-g-DMAE-BC to a bacterial suspension with KGM is plotted versus time. Upon addition of the KGM-g-DMAE-BC, the O.D. of the E. coli suspension at 260 nm increased. Increasing the amount of KGM-g-DMAE-BC also causes the ratio of O.D. to rise, suggesting that the leakage of DNA, RNA, and other materials correlates well with growth inhibition and a bacteriostatic effect (see Figure 2).
TTC test
Table 1 - Absorbance of TF and KGM and KGM-g-DMAE-BC after exposure for different periods of time*
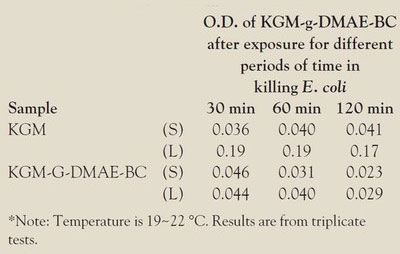
After incubation with KGM and KGM-G-DMAE-BC, E. coli is either present in the supernatant liquid or adheres to the surface of KGM and KGM-G-DMAE-BC (see Table 1). The bacterium that adheres to the surface of KGM and KGM-g-DMAE-BC is either alive or killed by the cationic group of KGM-g-DMAE-BC, which was demonstrated by the TTC test. The principle of the TTC test is that only live bacteria produce active D Hase. D Hase can transform TTC to TF, which can be quantified by UV-VIS absorbance (see Ref. 6). When E. coli is incubated with KGM, the quantity of TF in the liquid phase is higher than that in the solid phase (Table 1). This can be explained in one of two ways. One possibility is that KGM absorbs little bacteria or does not absorb at all. The other is that KGM is not bactericidal. On the contrary, in the KGM-G-DMAE-BC experiment, both the amount of live bacteria in the supernatant liquid and the bacteria adhering to the solid surface were very low, which suggests that the bacteria absorbed by KGM-G-DMAE-BC were killed by KGM-G-DMAE-BC.
Conclusion
This study proves that KGM-g-DMAE-BC is able to absorb E. coli to its surface and then killed the bacteria by destroying their membrane. These results also provide further evidence that KGM-g-DMAE-BC is a useful medicinal material for preventing infection.
References
- Ebi, N.; Imazato, S.; Noiri, Y. Dental Materials2001, 17, 485–91.
- Huang, R.; Du, Y.; Zheng, L.; Fan, L. Reactive & Functional Polymers2004, 59, 41–51.
- Xu, X.; Yang, Q.; Lu, A.; Li, Z.; He, Z.; Lin, F.; Zhang, T. Chemistry and Industry of Forest Products2006, 26, 75–8.
- Alef, K.; Nannipieri, P. Methods in Applied Soil Microbiology and Biochemistry; Academic Press: New York, NY, 1995; 228–9.
- Abbondanzi, F.; Cachada, A.; Campisi, T.; Guerra, R.; Raccagni, M.; Iacondini, A. Chemosphere2003, 53, 889–97.
- Chen, C.; Cooper, S. Biomaterials2002, 23, 3359–68.
The authors are with the College of Pharmacy, Zhengzhou University, 100 Science Rd., Zhengzhou, Henan 450001, China; tel./fax: +86 371 66658236; e-mail: [email protected]. This work was supported by the Nature Science Foundation of Zhengzhou City, Henan Province, China, no. 064SGBS33220-6.