FTIR spectroscopy has been widely used to evaluate the secondary structure and conformational changes of proteins.1–4 The amide I band (1600–1700 cm–1) is dominated by the C=O stretch vibration and is sensitive to protein secondary structure changes.3 The analysis of the secondary structure is normally performed by taking the second derivative of the original spectra to show the “fingerprint” of secondary structure features.5 A band at approximately 1632–1639 is present in globular and therapeutic proteins, such as IgGs, that are dominated by intramolecular β-sheets.3 The appearance of a band at 1616–1620 cm–1 is normally indicative of new intermolecular β-sheet formation that is often associated with protein denaturation.3
The attenuated total reflectance (ATR) technique has been commonly used in FTIR spectroscopy analysis.5,6 Analysis of protein samples using this technique can be performed in either a liquid or a dried state. ATR-FTIR spectroscopy has previously been used to study the structures of many globular proteins including bovine serum albumin (BSA) and IgGs on different silica surfaces.7 However, very limited work with protein-based virus-like particles were recognized during the authors’ studies.
This article describes the use of ATR-FTIR spectroscopy with a mercury cadmium telluride (MCT)/liquid nitrogen detector to evaluate the aggregation state of two different proteins: human papillomavirus (HPV) Type 6 L1 protein, and a mouse immunoglobulin (IgG). Both of these proteins are dominated by β-sheet structures. Following the temperature-induced aggregation of these proteins, samples were analyzed using the ATR-FTIR method. The method used in this study required only a small amount of material (approximately 2 μL). There was no requirement for deuterium oxide. A significant transition from a native intramolecular β-sheet to a nonnative intermolecular β-sheet was observed for both proteins during temperature stress treatment. This was indicated by the shift of the protein FTIR peaks and correlation to the temperature stresses. A peak at 1640 cm–1 progressively decreased and a new peak at approximately 1625 cm–1 was observed. The peak at 1640 cm–1 corresponds to a native intramolecular β-sheet, while the peak at 1625 cm–1 corresponds to a nonnative intermolecular β-sheet, and is indicative of aggregation. The corresponding particle sizes of samples generated at different temperatures were measured using dynamic light scattering (DLS). A plot of the second derivative peak height at 1625 cm–1 versus particle size indicated a strong correlation between the high-energy β-sheet formation at 1625 cm–1 and increased particle size (aggregation).
Methods
Sample preparation
Beta-sheet structure-dominated recombinant HPV Type 6 L1 protein virus-like particles (VLPs) (Bioprocess R&D, Merck & Co., Inc., West Point, PA) and mouse IgG samples (Sigma, St. Louis, MO) at concentrations of 1 mg/mL were dialyzed in 150 mM NaCl, pH 7.0, then heat-stressed at various temperatures for 1 hr to induce different levels of aggregation. The heating process was stopped by quenching on ice.
FTIR spectroscopy was performed with a PerkinElmer (Llantrisant, Wales, U.K.) FTIR instrument using ATR methods and an MCT/liquid nitrogen detector. Two microliters of sample were prepared on the ATR surface, dried with air, and then measured at room temperature. The zero and second-derivative spectra were obtained using the computer software. The second-derivative spectra were plotted for each temperature point.
DLS was performed using 50 μL of sample on a Malvern Nanosizer (Worcestershire, U.K.) with a 45-μL cuvette at room temperature. Each of the results shown is an average of two tests performed with a total of five replicates each.
Results and discussion
The HPV Type 6 L1 protein VLP sample was incubated at a starting temperature of 25 °C, which was followed by 1-hr incubations of individual samples at elevated temperatures up to 95 °C. A sample maintained at 4 °C was used as a control. Following ATR-FTIR spectroscopy, the second-derivative spectra were plotted for each temperature point (Figure 1a). The results indicated the presence of a peak originating at approximately 1640 cm–1 and progressively shifting to 1625 cm–1 (Figure 1a). The peak at 1625 cm–1 corresponds to a nonnative high-energy intermolecular β-sheet, which is indicative of aggregation. The transition from a native state to an aggregated state was initiated between 50 and 60 °C (Figure 1a). The change in protein structure that occurs between 50 and 60 °C corresponds to the first transition peak that is observed during the temperature-induced unfolding of HPV Type 6 L1 protein VLPs using differential scanning calorimetry (DSC).8
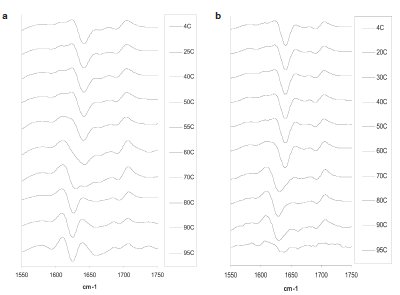
Figure 1 - Second-derivative spectra of proteins measured following temperature-induced aggregation processes. a) HPV Type 6 L1 protein particles, b) mouse IgG.
Mouse IgG samples were incubated at an initial temperature of 20 °C, followed by 1-hr incubation at temperature intervals up to 95 °C. A sample maintained at 4 °C was used as a control. The results of the study are depicted in Figure 1b. The transition of a peak at 1640 cm–1 to a peak at 1625 cm–1 was observed (Figure 1b). The change from a native to an aggregated state was initiated between 50 and 70 °C.
Particle size and correlation to FTIR spectroscopy
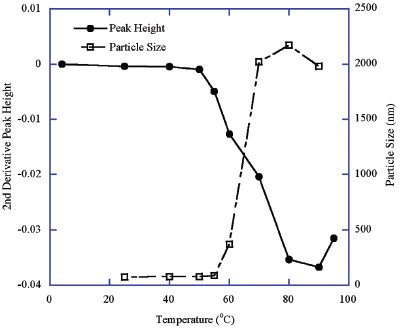
Figure 2 - Temperature-induced aggregation of HPV Type 6 L1 protein VLPs: FTIR peak height difference versus particle size change.
The particle sizes of the temperature-stressed HPV Type 6 L1 protein samples were measured using DLS. A plot of the stress temperature versus particle size and FTIR peak height at 1625 cm–1 is depicted in Figure 2. These results indicate a progressive increase in the particle size as the temperature increased with a concomitant change in the protein FTIR peak position. A dramatic particle size increase occurred between 55 and 70 °C, which is consistent with the change in FTIR peak position between 50 and 80 °C (Figures 1a and 2). It was noted that the transition of the FTIR peak occurs over a temperature range wider than that of the particle size change, which is expected from a complex protein assembly, and the aggregation occurs after protein conformational changes or structural collapse. This can be better explained based on the structure of HPV VLPs, which are composed of pentameric structures consisting of individual HPV L1 monomers. A small fraction of VLP structural changes would result in the initiation of aggregation. As a result of the ongoing aggregation, an increase in the apparent particle size of the protein would be detected by the light scattering method since the larger particles scatter more light per unit of mass. As a result of protein conformational or VLP structural change, an increase in the FTIR peak at 1625 cm–1 and a decrease in the FTIR peak at 1640 cm–1 occur. This is due to the transition from the intramolecular β-sheet structure of the native protein to the intermolecular β-sheet structure of the aggregated protein.
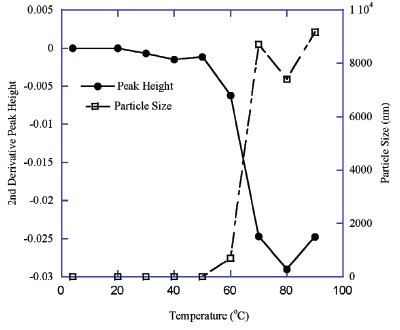
Figure 3 - Temperature-induced aggregation of mouse IgG: FTIR peak height difference versus particle size change.
Very similar results were obtained for the mouse IgG sample using DLS and FTIR to analyze the protein particle size and secondary structure. A plot of the temperature versus particle size and FTIR peak height at 1625 cm–1 are depicted in Figure 3. The intrinsic particle size of IgG molecules (approximately 10–12 nm) is significantly smaller than that of the HPV VLPs (approximately 50–70 nm) (Figures 2 and 3). However, the temperature-induced aggregates of both samples all have particle sizes largely beyond these ranges, which results in values outside of the DLS range of 1000 nm. Again, the FTIR peak shift and particle size change of the IgG molecules also occurred at the same temperature range of 50 to 70 °C (Figure 3). It was also observed that a possible small pretransition in the FTIR peak at 1625 cm–1 occurred beginning at 30 °C. However, this possible transition was not associated with aggregation or increased particle size (Figure 3).
Conclusion
The studies described in this paper demonstrated a correlation between an FTIR peak generated at approximately 1625 cm–1 and increased particle size. The use of ATR-FTIR spectroscopy offers a rapid method for identifying aggregation using a small sample size.
References
- Manning, M.C. Use of infrared spectroscopy to monitor protein structure and stability. Expert Rev. Proteomics 2005, 2, 731–43.
- Byler, D.M.; Susi, H. Examination of the secondary structure of proteins by deconvoluted FTIR spectra. Biopolymers 1986, 25, 469–87.
- van Stokkum, I.H.M.; Linsdell, H.; Hadden, J.M.; Harris, P.I.; Chapman, D.; Bloemendal, B. Temperatureinduced changes in protein structures studied by Fourier transform infrared spectroscopy and global analysis. Biochemistry 1995, 34, 10,508–18.
- Bramanti, E.; Benedetti, E. Determination of the secondary structure of isomeric forms of human serum albumin by a particular frequency deconvolution procedure applied to Fourier transform IR analysis. Biopolymers 1996, 38, 639–53.
- Buijs, J.; Norde, W.; Lichtenbelt, J.W.Th. Changes in the secondary structure of adsorbed IgG and F(ab′)2 studied by FTIR. Langmuir 1996, 12, 1605–13.
- Ball, A.; Jones, R.A.L. Conformational changes in adsorbed proteins. Langmuir 1995, 11, 3542–8.
- Giacomelli, C.E.; Bremer, M.G.E.G.; Norde, W. ATR-FTIR study of IgG adsorbed on different silica surfaces. J. Colloid Interface Sci. 1999, 220, 13–23.
- Shank-Retzlaff, M.L.; Zhao, Q.; Anderson, C.; Hamm, M.; High, K.; Nguyen, M.; Wang, F.; Wang, N.; Wang, B.; Wang, Y.; Washabaugh, M.; Sitrin, R.; Shi, L. Evaluation of the thermal stability of Gardasil. Human Vaccines2006, 2, 147–54.
Dr. Meyer is Research Fellow, Dr. Lu is Senior Research Biochemist, Mr. Mach is Senior Investigator, and Dr. Shi is Associate Director, Biologics and Vaccines, Pharmaceutical Research and Development, Merck & Co., 770 Sumneytown Pike, P.O. Box 4, West Point, PA 19486-0004, U.S.A.; tel.: 215-652-3992; fax: 215-652-5299; e-mail: [email protected].