The cardinal principle for an operation involving chemical agents is to limit the potential exposure to a minimum number of personnel, for a minimum period of time, to a minimum amount of the chemical agent consistent with safe and efficient operations.1 This requires that the synthetic schemes be rapid and free from unnecessary by-products that would require otherwise further sample handling. Thus, acid/base chemistries with alcohols as intermediates are often used to synthesize phosphonofluoridates, phosphoramidocyanidate, and phosphonothioates.
Dovetailing into the cardinal principle is the need for a rapid chemical analysis method that can accurately confirm the identity and purity of the starting materials, intermediates, and final product(s). In order to accomplish these objectives, the U.S. Army’s Edgewood Chemical Biological Center (ECBC) has been using the Direct Analysis in Real Time (DART™) method (JEOL USA, Inc., Peabody, MA) since 2002.2 Since that time, a flurry of other open-air methods based on the use of metastable species has been seen.
During the course of a large synthesis project comprising many organophosphorus compounds and their isotopically labeled analogs, it was observed that the DART datum for labeled alcohol intermediates neither corroborated the nuclear magnetic resonance (NMR) datum nor the GC-MS datum. Yet the data from these three analytical methods were in agreement when the final chemical agent product was analyzed. An investigation was initiated in order to discover the cause of this discrepancy, and a procedure was developed that allowed alcohols and organophosphorus intermediates to be analyzed by DART.
Experimental
Chemicals were either synthesized in-house or purchased from Aldrich Chemical Co. (Milwaukee, WI). The alcohol precursors were converted into their corresponding carbamates using the N-hydro-C-alkoxyaddition reaction. The reaction occurs at room temperature without by-product formation and is simple to perform. The alcohol was pipetted (10–50 μL) into a small vial, weighed, 1000 μL of hexane was added, and then a 1.1 molar excess of phenyl isocyanate or substituted phenyl isocyanate was introduced. Because the O-alkyl carbamate product is not soluble in hexane, it is automatically purified as it crystallizes out of solution. An additional benefit of the reaction scheme is that crystal formation conveniently indicates the end-point of the reaction.
The experimental apparatus comprised a model 100 DART ion source (serial number 001-JL1) and a modified JEOL AccuTOF mass spectrometer (model JMS-100TLC). The DART source consists of a tube divided into three chambers. A needle in the first chamber is electrified to a few kilovolts to form a plasma that produces helium cations, electrons, and excited-state species, which are the working reagent in the DART method. The second chamber has an electrode to remove most of the ions from the gas stream. The last chamber consists of a grid that faces a mass spectrometer sampling orifice. Samples were analyzed by dipping a closed-end glass capillary (1 × 90 mm) onto the sample crystals at the bottom of the reaction vial, and holding the capillary in open air between the DART grid and AccuTOF sampling orifice. The gas flow and electrode potentials have a broad range of operating values that are not critical to the information fidelity of the experiment. Many combinations of settings gave similar sensitivity.
Mass spectra were recorded using the modified time-of-flight mass spectrometer, operating at 6000 mass resolution (FWHM definition). The sampling orifice was operated at 60 V relative to ground in order to attract the analyte ions into the spectrometer. A dilute nicotine solution provided a lock mass correction. Data were processed on modified manufacturer software.
Results and discussion
Since 2002, it has been the authors’ experience that MS analysis by DART results in clutter-free spectra of stockpile and nonstockpile chemical warfare agents, explosives, narcotics, and toxins. Mass spectra typically consist of a single mass peak for each component present in the sample. This allows for easy spectral interpretation along with unambiguous mass assignment, and is particularly valuable for the analysis of mixtures. Therefore, the authors were initially surprised to find that alcohols of high purity gave a large number of spectral peaks.
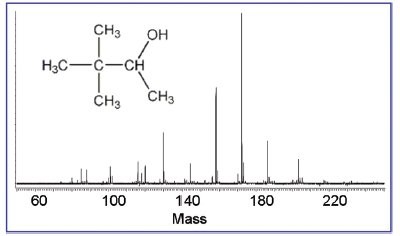
Figure 1 - Mass spectrum of pure pinacolyl alcohol, synthesized at the Edgewood Chemical Biological Center (Aberdeen Proving Ground, MD).
The DART mass spectrum of pinacolyl alcohol (Figure 1) contained numerous peaks beyond the molecular weight of the neutral. This result was unforseen and unlike the behavior of other compound classes. It was puzzling because the NMR and GC-MS spectra indicated a pure reagent. Yet when this same alcohol was reacted to produce pinacolyl methylphosphonofluoridate (Agent GD), the mass spectrum indicated formation of a pure product with little fragmentation (Figure 2). The DART datum now concurred with the NMR and GC-MS data. This was confirmed by the close agreement between the measured mass of the (MH+NH3)+ molecular cation and its expected mass, 200.1219 versus 200.1216 Da.
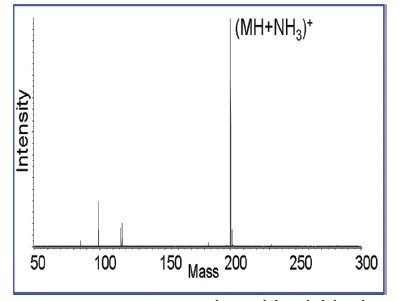
Figure 2 - Mass spectrum of pinacolyl methylphosphonofluoridate (Agent GD). The measured mass of the (MH+NH3)+ molecular cation agrees well with its expected mass, 200.1219 versus 200.1216 Da. A few diagnostically useful fragment ions were induced by raising the orifice voltage.
Initially, no pattern of similarity emerged among the different alcohols used in chemical agent synthesis. Each alcohol appeared to behave in its own way. A decision was made to examine a wider variety of alcohols in order to gather enough data to gain a better understanding of the mechanism. The alcohols examined were branched, unbranched, aromatic, saturated cyclic, linear alcohols with double bonds, phenolic, bi-cyclo, and nitrogen containing. Exact mass measurements of the major peaks in the mass spectrum were made in order to assign correct and unambiguous elemental compositions to the unexpected mass spectral peaks. The average mass accuracy for all the peaks of all the alcohols was found to be ±0.00004 Da by summing the mass deviations.
An unexpectedly large number of peaks were observed in the mass spectrum of each alcohol (Table 1, column 2 and supplemental materials). The overall finding is that alcohols undergo oxidation by a variety of mechanisms when ionized by DART. These include loss of multiple hydrogen molecules, addition of oxygen atom, loss of water, loss of methylene, and loss of hydrogen. Other compound classes (chemical warfare agents, narcotics, or explosives) do not exhibit these adducts and fragmentations. DART consistently produces a clean mass spectrum consisting of just the MH+ molecular cation. Proton transfer is the most common mechanism for positive-ion formation. The helium 23S state rapidly reacts with atmospheric water (reaction 1) with a measured reaction cross-section3 of 100 Å.2 Water ion/molecule reactions result in the formation of hydronium ions (reaction 2). Proton transfer from the hydronium ions to the analyte (reaction 3) is the predominant reaction mechanism for the formation of positive ions in DART. Sometimes an (MH+NH3)+ molecular cation is observed by the propinquity of the human body from which ammonia is a natural by-product.
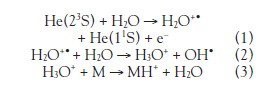
There were two exceptions to these general findings: 3-quinuclidinol and diisopropylamino alcohol, both of which produced intense MH+ molecular cations as the base peak (Table 1 and supplemental materials for spectra). The nitrogen atom in these two alcohols increases their proton affinity; thus, when the ionizing proton is captured, a cation of greater stability results. These data are consistent with the suggestion that increasing the nucleophilicity of the alcohol increases the rate of acylation.4
Based on the observation that nitrogen atom-containing alcohols gave intense MH+ molecular cations, a hypothesis was conceived that could be tested: Alcohols add to phenyl isocyanate to form carbamates via an N-hydro-C-alkoxy-addition reaction (reaction 4), and since the proton affinity of the carbamate is more than its corresponding alcohol, intense MH+ cations, without oxidation by-products, should result.
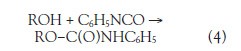
When this experiment was performed, fewer mass spectral peaks were observed (Table 1, column 3 and supplemental materials). The method is significant because it is a one-pot reaction that occurs at room temperature, it is tolerant of moist conditions, the reaction yield is high and is catalyzed by light, and the product is automatically purified as it crystallizes out of solution.
The authors’ method has three beneficial consequences. It simplifies the spectral interpretation of unknown alcohols; it concentrates the analyte ion signal into fewer data channels, thereby increasing analytical specificity; and a 20-fold detection sensitivity enhancement is seen. Nearly every alcohol gave an MH+ or (MH+NH3)+ molecular cation as the base peak. An oxygen adduct was seen for citronellyl phenyl carbamate and eugenyl phenyl carbamate, but these alcohols are not relevant in chemical agent synthesis and are of no present consequence. These data, taken as a whole, support the authors’ hypothesis.
Other carbamates were produced from alkyl isocyanates to search for the most suitable chemistry when analyzing branched, hindered alcohols relevant in chemical agent synthesis. Pinacolyl alcohol was chosen because it is a precursor for pinacolyl methylphosphonofluoridate. None of the alkyl isocyanates were as suitable as phenyl isocyanate. Pinacolyl ethyl carbamate was a gooey gel. Pinacolyl isopropyl carbamate gave an (alcohol + ammonia) adduct, and pinacolyl-2-chloroethyl carbamate and pinacolyl-2-bromoethyl carbamate gave a cluster of molecular ion isotopes.
Other solvents were investigated as well. Carbon tetrachloride was tried since it is known for its ability to form a charge–transfer complex.5 The reaction produced a single peak as did hexane as a solvent, but the carbamate product was a viscous fluid instead of neat crystals.
Conclusion
The premise of the DART design is that proton transfer is the most common mechanism for positive-ion formation, which produces an uncluttered mass spectrum consisting of the MH+ molecular cation. But since samples are ionized in the open air under ambient conditions, in situ oxidation gives a plethora of ionic species when the analyte is an alcohol. This limitation can be eliminated by converting the alcohol to its corresponding carbamate using the N-hydro-C-alkoxy-addition reaction.
References
- The Army Chemical Agent Safety Program; Shinseki, E.K., General U.S. Army Chief of Staff; Army Regulation 385–61, Nov 2001; Chap. 2–4a.
- Laramée, J.A.; Durst, H.D.; Connell, T.R.; Nilles, J.M. Detection of chemical warfare agents on surfaces relevant to homeland security by direct analysis in real-time spectrometry. Am. Lab.2008, 40(16), 16–20.
- Cold Collisions of Metastable Helium Atoms; Maastwijk, H.C.; Ph.D. thesis: University of Utrecht, The Netherlands, 1997.
- Satchell, D.P.N.; Satchell, R.S. Acylation by ketens and isocyanates: a mechanistic comparison. Chem. Soc. Rev. 1975, 4, 231–50.
- Foster, R.; Organic Charge–Transfer Complexes; Academic Press: New York, NY, 1969; pp 278–80.
Dr. Laramée is Principal Scientist, Scientific Applications International Corp. (SAIC), Box 68, Aberdeen Proving Ground, MD 21010, U.S.A.; tel.: 410-436-2987; fax: 410-436-7317; e-mail: [email protected]. Dr. Durst is Research Chemist and Head, Chemical Methodology Team, U.S. Army Edgewood Chemical Biological Center, Aberdeen Proving Ground, MD. Dr. Nilles and Ms. Connell are Senior Scientists, Science & Engineering Technologies, Inc., Springfield, VA.The authors gratefully acknowledge the facility support of the Research & Technology Directorate at the Edgewood Chemical Biological Center (ECBC) and fiscal support from the Agent Fate Program (DTO.42), BA07RAS101, by the Joint Science and Technology Office (JSTO) in the Defense Threat Reduction Agency (DTRA), and the DoD Chemical and Biological Defense Program. A long overdue thanks is owed to William Creasy (SAIC), who in 2002 kindly brought to the authors’ attention Ref. 3, which gives the cross-section for the reaction of metastable helium with water. This document is UNCLASSIFIED and cleared for public release through American Laboratory.