Chirality is an intrinsic property of living matter, and many biological molecules exhibit a preference for one enantiomer over another. As such, chirality has been introduced into more than half of the approved drugs worldwide.1 Enantiomers can have differing biochemical effects. In order to market drugs with better potency and safety profiles, the pharmaceutical industry has moved toward advancing single enantiomer drugs over racemates, making absolute stereochemistry determinations an essential part of the drug discovery process.
X-ray diffraction continues to be the gold standard for absolute configuration determinations of chiral compounds. In some cases, though, it is difficult to obtain single crystals of sufficient quality for X-ray analysis, particularly in drug discovery, where intermediates and precursors can exist as liquids or oils and are challenging to crystallize. These limitations can cause significant delays in research efforts focused on chiral drug candidates.
Vibrational circular dichroism (VCD) has gained great momentum as an alternative to X-ray diffraction for reliably determining the absolute configurations of chiral molecules, and has quickly found a place in drug discovery. The method consists of comparing the results of a VCD spectrum of a sample in solution to the results of an ab initio or density function theory (DFT) calculation. If the major bands of the measured and calculated VCD spectra agree in relative magnitude and sign, then the absolute configuration of the sample is assigned that of the enantiomer used in the calculation. If the relative magnitude agrees, but the signs are opposite, then the sample is assigned the opposite stereochemistry of the enantiomer used in the calculation. Indeterminate situations arise when there is not good agreement between calculated and observed VCD transitions.
Instrumentation
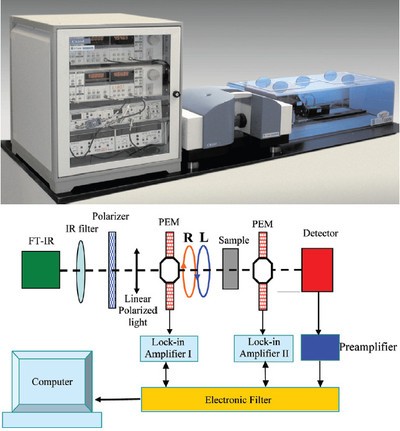
Figure 1 - ChiralIR spectrometer with DualPEM (top) and instrument schematic (bottom).
The ChiralIR™ spectrophotometer with DualPEM™ dual photoelastic modulator (PEM) (BioTools, Inc., Jupiter, FL) was used in this study. The instrument features PEMs before and after the sample cell for better baseline and elimination of major linear birefringence artifacts. The IR light beam from the interferometer is redirected to a side port. The ZnSe photoelastic modulator and detector assembly that includes the focusing lens are configured on a rail system, with the addition of a second ZnSe modulator. Each modulator is referenced to its own lock-in amplifier and its respective signals subtracted in real time (Figure 1). Samples are usually measured in a 100-µm pathlength cell with concentrations of about 20 mg/mL. In general, VCD spectra are measured in solution using solvents like CCl4, CDCl3, and DMSO-d6, and typically take 8 hr to acquire.
Principles of absolute configuration assignment
Since determination of absolute chirality involves comparing the results of a solution-phase VCD measurement to the results of an ab initio or DFT calculation for one of the enantiomers, the accuracy and reliability of these calculations are as important as the VCD measurement itself. Even with a computationally sound algorithm, other factors can influence the calculated results and should be taken into consideration where appropriate. For example, solute–solvent and solute–solute interactions can greatly affect the conformation of molecules in solution and should be considered when generating calculated VCD spectra.2 In some cases, solvent selection can affect the absolute configuration assignment process. For instance, CDCl3 can form weak hydrogen bonds with electron-rich atoms in the compounds being analyzed,3 and DMSO-d6 can disrupt intramolecular or intermolecular hydrogen bonds. For some molecules, hydrogen bonding and other nonbonded interactions can stabilize the lowest-energy conformer. However, such interactions can be difficult to predict and can therefore complicate the computational phase of VCD analysis. Further, it is not practical to consider all possible interactions for every sample; thus care should be taken to simplify and properly model the major ones that will have an effect on the lowest-energy conformers. In this manner, calculations that better resemble the experimental data can be obtained within a reasonable time.
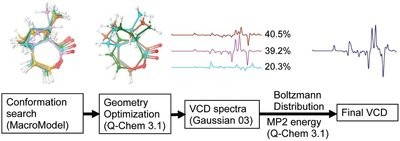
Figure 2 - Process flow for generating calculated VCD spectra.
Another factor to consider is time. In the fast-paced pharmaceutical drug discovery environment, throughput and turnaround time are critical. Since the computational resources for ab initio calculations are significant, it is important to select algorithms that can generate reliable results within a reasonable time frame (no more than a couple of days). Figure 2 shows a flow chart of a typical calculation routine. The Q-Chem software package (Q-Chem, Inc., Pittsburgh, PA) was used for geometry optimization. MP2 energy was also calculated using Q-Chem when needed. The Gaussian03 software package (Gaussian, Inc., Wallingford, CT) was used for frequency and VCD calculations.
Application example
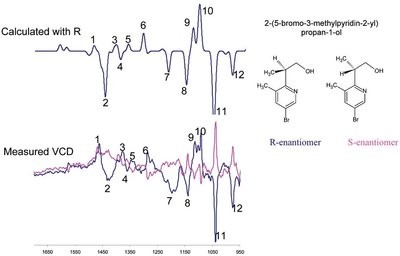
Figure 3 - Calculated and measured VCD spectra of 2-(5-bromo-3-methylpyridin-2-yl)propan-1-ol.
The commercial availability of reliable and relatively fast algorithms for ab initio calculations of VCD spectra, combined with the vastly improved performance of modern computers, has launched absolute stereochemistry assignments by VCD beyond the arena of pure exploratory or academic research into new areas such as pharmaceutical drug discovery and development. An example of absolute stereochemistry by VCD using enantiomers of 2-(5-bromo-3-methylpyridin-2-yl)propan-1-ol is presented. In this example, the calculated R-conformer contained an intramolecular hydrogen bond between the hydroxyl proton and pyridine nitrogen restricting the rotation of the propanol group. The resulting calculated VCD spectrum of this R-conformer and the experimental spectra for both enantiomers are shown in Figure 3. The VCD bands of the R-enantiomer (shown in blue) are in good agreement with the calculated bands, allowing confident assignment of absolute stereochemistry.
Discussion and conclusion
VCD offers a new and powerful approach for the absolute stereochemistry determinations of chiral molecules in solution, and is finding increasing applications in the pharmaceutical industry, especially in drug discovery. Continuous advancements of commercial VCD spectrometers enable users to acquire VCD spectra in short periods of time with minimal artifacts. Combined with the advancements of computation hardware and algorithms, VCD is poised to deliver results in-line with the demands of a fast-paced drug discovery environment. Not all samples are amenable to VCD analysis, however. From the spectroscopic side, conformationally flexible molecules can prove challenging due to poor experimental VCD signal. Indeed, such flexibility also makes crystallography difficult. Also, molecules with extensive nonbonded interactions can be computationally challenging. However, there are numerous instances in which VCD can be successfully applied, and in the future it is expected that this will be a very active field of analytical science.
References
- Caner, H.; Groner, E.; Levy, L. Trends in the development of chiral drugs. DDT2004, 9(3), 105–10.
- Izumi, H.; Ogata, A.; Nafie, L.A.; Dukor, R.K. A revised conformational code for the exhaustive analysis of conformers with one-to-one correspondence between conformation and code: application to the VCD analysis of (S)- ibuprofen. J. Org. Chem.2009, 74(3), 1231–6.
- Debie, E.; Bultinck, P.; Herrebout, W.; van der Veken, B. Solvent effects on IR and VCD spectra of natural products: an experimental and theoretical VCD study of pulegone. Phys. Chem. Chem. Phys.2008, 10, 3498–3508.
The authors are with Pharmaceutical Candidate Optimization, Bristol-Myers Squibb Co., 311 Pennington-Rocky Hill Rd., Pennington, NJ 08534, U.S.A.; tel.: 609-818-4110; fax: 609- 818-3450; e-mail: [email protected]. The authors thank Dr. Scott Priestley and Dr. Nicholas Wurtz of Bristol-Myers Squibb Co. for providing the 2-(5-bromo-3-methylpyridin-2-yl)propan-1-ol sample and for their helpful comments. They also thank Mr. Atsu Apedo of Bristol-Myers Squibb Co. for performing chiral purification on the sample.