Understanding and controlling the characteristics of proteins in solution is fundamental to predicting their aggregation behavior and determining appropriate conditions for storage, crystallization, and other processing parameters—essential in areas from academic research to the manufacture of an increasing number of protein-based products. One powerful tool widely used in characterizing dispersions or solutions of proteins is light scattering, which encompasses techniques for measuring both size and zeta potential. This article describes a measurement cell that enables determination of zeta potential in high-concentration, low-volume samples without dilution, and examines its significance.
Light scattering
Light scattering in general is a powerful tool for characterizing dispersions or solutions of proteins.1 Absolute molecular weight is accessible using static light scattering (SLS), diffusion coefficient and therefore hydrodynamic size by dynamic light scattering (DLS), and information relating to surface charge by electrophoretic light scattering (ELS).
In this work, ELS was used to measure the electrophoretic mobility (EPM) and derive the zeta potential—the potential at the surface of shear where the molecule and its associated sheath of counter ions slip through the solution under the action of the applied electric field.2,3 This differs from the surface potential because of the presence of ions between the surface and the free solution. However, in the absence of specific absorption that effectively modifies the surface, it is generally proportionally related, and is important because it is this potential that governs electrostatic molecular interactions.
Measuring small-molecule proteins
Proteins become charged in solution through ionization of the carboxyl and amine groups present in the various amino acids making up the molecule.4 Lysozyme, used in the study described below, is a readily available serum protein—an enzyme containing 129 amino acids that is found in many bodily fluid secretions. It is a natural bactericide first identified as such by Alexander Fleming in 19225 that functions by cleaving the polysaccharide chains forming the bacterial cell wall.6 Lysozyme is an ellipsoid approximately 3.3 × 5.5 nm in size with a molecular weight of 14.7 kDa.7 This means that it is relatively small in terms of a subject for ELS measurements.
The directed motion of ELS is “smeared out” to an extent by the random diffusion of Brownian motion, and the diffusion coefficient for such a small molecule is large (10–10 m2/sec). In addition, since light scattering varies with the sixth power of radius for molecules much less than the illuminating wavelength, the scattering signal is comparatively small. In this work, the weakness of scattering was compensated for by preparing samples at reasonably high concentrations.
Measuring at high concentration
In ELS detection, minimum and maximum sample concentration measurement limits depend on particle size, polydispersity of particle distribution, and the optical properties of particles in the sample. As described by the Beer-Lambert law, the transmission of light through a substance is logarithmically dependent on both the absorption coefficient of the substance and the distance the light travels through the material (i.e., the pathlength). The absorption coefficient is a product of the concentration c of absorbing species in the material and an intrinsic property of the material called the molar absorptivity, which, in turn, depends on particle size.
While more traditional capillary cells used for zeta potential measurement have a pathlength of 4–10 mm, for example, the high concentration cell for the Zetasizer Nano system (Malvern Instruments, Malvern, Worcestershire, U.K.) has a pathlength of only 1.5 mm. By reducing the distance the light has to travel through the sample, the cell is able to deliver zeta potential measurements for highly concentrated, less transparent samples. The cell also requires only small volumes of sample down to as little as 200 µL.
Investigating dependence of zeta potential on pH
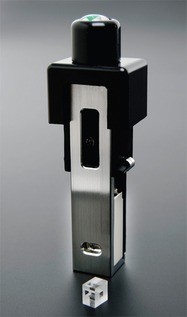
Figure 1 - High-concentration/low-volume zeta potential cell with quartz capillary in foreground.
Lysozyme was chosen to illustrate the capability of the high-concentration cell because measuring its zeta potential is challenging. It has also been the subject of a significant amount of research and is readily available. In this work, lysozyme derived from chicken egg white (99% pure) (Sigma-Aldrich, St. Louis, MO) was used. The aim of the measurements was to investigate the dependence of zeta potential on pH. This was adjusted manually by adding either 0.025 M NaOH or 0.025 M HCl to around 5 mL dispersion of 20 mg/mL lysozyme in approximately 0.6 mM NaCl.
Zeta potential measurements were made using a Zetasizer Nano ZS equipped with the high-concentration/low-volume zeta potential accessory (Figure 1). The small sample volume of the cell allowed just 200 µL of material to be used for each measurement. Manual titration was performed over a pH range from 3.03 to 9.43, with three repeat measurements at each pH point. All measurements were made at 25 °C. An electric field of 25 V/cm was applied.
A sample of the stock solution was measured by DLS at 25 oC, and the main size population was found to be at a hydrodynamic diameter of around 4.0 nm, as expected for lysozyme.
Reducing surface charge effects
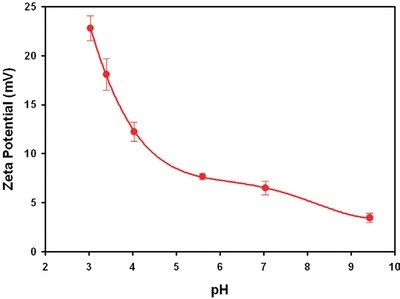
Figure 2 - Plot of the zeta potential values obtained for lysozyme prepared at different pH values.
Table 1 - Zeta potential results obtained for lysozyme samples prepared at different pH values
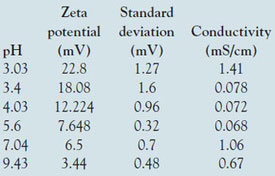
The zeta potential results obtained for lysozyme in the high-concentration cell are summarized in Table 1 and plotted in Figure 2. The table shows the zeta potential means (in mV), the standard deviation (in mV) for the three repeat measurements taken on each sample, the measured conductivities (in mS/cm), and the pH of each sample. Note that the sign of the zeta potential is positive across the range covered and decreases steadily in magnitude as the pH increases. This is to be expected since the isoelectric point (IEP, the point of zero zeta potential) is known to be at approximately pH 11.8,9 The values between pH 3.5 and 4.5 also agree with published data.10 Note, however, that there is a subtlety here. The point of zero zeta potential may differ from the point of zero charge if absorption of ions to the molecule from solution occurs. Negative values of EPM in the pH range 6–10 have been reported.11
This is an indication that in those measurements, some available anionic species adsorbed to the lysozyme surface. It is generally accepted that measurements on cationic species are challenging simply because many moieties that are present, even in small concentrations, are likely to be negatively charged and attracted to a positive surface. These include traces of surfactants used in cleaning, for example. Performing the measurements at a relatively high concentration can help avoid these effects.
Table 2 - Zeta potential results obtained for lysozyme samples prepared at different pH values in 0.15 M NaCl
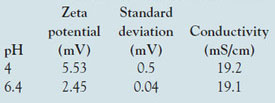
Measurements were also conducted at higher ionic strength (nominally 0.15 M NaCl) over a limited pH range. The values obtained are summarized in Table 2. The reduction in magnitude of the zeta potential by higher salt is an example of ionic screening; the ion atmosphere effectively attenuates the effect of the surface charge without actually adsorbing to the surface. A similar trend of decreasing value of zeta potential as pH is increased was observed.
Conclusion
Zeta potential measurements of lysozyme exploiting the low-volume capability of a high-concentration zeta potential cell were presented as a function of pH and salt concentration. The values obtained in this study are in agreement with published data. Using the high-concentration/low-volume zeta potential cell with the Zetasizer Nano ZS, ELS measurements were unrestricted by previous concentration limits. By reducing the optical path, the cell permits the system to maintain the levels of transmission necessary to deliver accurate results for small-molecule proteins at higher concentrations and lower volumes.
References
- Berne, B.J.; Pecora, R. Dynamic Light Scattering: With Applications to Chemistry, Biology, and Physics; Dover Publications: Mineola, NY, 1976.
- Hunter, R.J. Zeta Potential in Colloid Science: Principles and Applications; Academic Press: London, U.K., 1988.
- Delgado, A.V.; González-Caballero, F.; Hunter, R.J. Measurement and interpretation of electrokinetic phenomena. Pure Appl. Chem.2005, 77, 1753–1805.
- Stryer, L. Biochemistry, 5th ed. W.H. Freeman & Co.: New York, NY, 2002.
- Fleming, A. Proc. Roy. Soc. Ser. B1922, 93, 306–17.
- Meyer, K.; Palmer, J.W.; Thompson, R.; Khorazo, D. J. Bio. Chem. 1935, 113, 479–86.
- Blake, C.C.F.; Koenig, D.F.; Mair, G.A.; North, A.C.T.; Phillips, D.C.; Sarma, V.R. Nature1965, 206, 757–61.
- Price, W.; Tsuchiya, F.; Arata, Y.J. Am. Chem Soc.1999, 121, 11,503–12.
- Green, R.J.; Su, T.J.; Lu, J.R. J. Phys. Chem. B2001, 105, 9331–8.
- Bernardo, A.; Calmanovici, C.E.; Miranda, E.A. Crystal Growth & Design 2004, 4, 799–805.
- Pérez-Rodríguez, M.; Prieto, G.; Rega, C.; Varela, L.M.; Sarmiento, F.; Mosquera, V. Langmuir1998, 14, 4422–6.
The authors are with Malvern Instruments Ltd., Enigma Business Park, Grovewood Rd., Malvern, Worcestershire WR14 1XZ, U.K.; tel.: +44 (0) 1684 892456; fax: +44 (0) 1684 892789; e-mail: [email protected].