Virus-like particles (VLPs) are of growing interest in the pharmaceutical sciences. During the past few years, they have gained more and more attention as a new, safe class of vaccines, suitable for the prevention of virus-induced infectious diseases1 and for the therapeutic treatment of chronic diseases2 and drug addiction.3 For the development of VLP-based pharmaceutical products, the application of reliable analytical tools is of great importance. The analytical method needs to be sufficiently sensitive to detect and quantify even small differences between active pharmaceutical ingredient (API) bulk materials and various formulations upon manufacture and storage. It has been demonstrated that the separation and subsequent quantification of different VLP species (fragments, monomers, dimers, trimers, and aggregates) is possible using asymmetric flow field-flow fractionation (AF4). This study illustrates an improved AF4 method for the investigation of VLP. Disadvantages such as long equilibration and analysis times, as well as the need for large sample amounts and eluent volumes, have been overcome by using new shortened channel geometries.
Field-flow fractionation
Field-flow fractionation (FFF) is a separation technique used to characterize molecules by size. It is a chromatographic-like process that relies on differences in a molecule’s laminar flow.4 In terms of separation range, selectivity, and resolution, it is a powerful, versatile separation method for high-molecular-weight molecules and particles. FFF comprises a family of techniques that have demonstrated, over more than two decades, the ability to characterize supramolecular species in a size range spanning many orders of magnitude, from macromolecules to micron-sized particles.5
Asymmetric flow field-flow fractionation is the most widely used and versatile FFF technique, providing a powerful analytical separation method for the characterization of biopolymers and bioparticles. Over the past few decades, AF4 has gained more and more attention as the FFF technique of choice and has been successfully applied for the analysis of the size and distribution of monoclonal antibodies,6 liposomes,7 lipid/DNA complexes,8 nanoparticles,9 and viruses.10 Furthermore, by coupling the technique with a multiangle laser light-scattering (MALLS) detector, it is possible to obtain the molecular weight distributions of the fractionated species.10
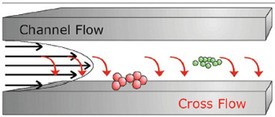
Figure 1 - Separation principle of AF4.
AF4 analysis is performed in a hollow channel (see Figure 1). The channel consists of two plates separated by a spacer foil. The upper channel plate is impermeable. The bottom channel plate, on the other hand, is permeable, and is made of a porous frit material. An ultrafiltration membrane, with a certain molecular-weight cutoff, covers the bottom plate to prevent the sample from penetrating the channel.
High-resolution separation is achieved within a very thin parabolic channel flow against which a perpendicular cross-flow is applied. Within the flow channel, the parabolic flow profile is created because of the laminar flow of the liquid: The stream moves slower closer to the boundary edges than it does at the center of the channel flow. When the perpendicular force field is applied to the flowing, laminar stream, the analytes are driven toward the boundary layer, the so-called “accumulation wall” of the channel. In turn, diffusion associated with Brownian motion creates a counteracting motion. Smaller particles, which have higher diffusion rates, tend to reach an equilibrium position higher up in the channel, where the longitudinal flow is faster. Thus, the velocity gradient flowing inside the channel separates different sizes of particles. The smaller particles are transported faster along the channel than the larger particles. This results in the smaller particles eluting before the larger ones.
The separation process requires three steps. During the first two steps—injection and focusing—the main flow is split, enters the channel from both ends, and is balanced to meet under the injection port. At this point, the flow moves down and exits the channel via the ultrafiltration membrane. When the sample is injected, it is focused in a thin band and concentrated toward the membrane. After complete transfer of the sample volume, the injection flow is stopped, and one typically allows for another minute of focusing before the flow pattern is switched to the elution mode. Now the flow enters only from the inlet port. The channel flow exits at the outlet, which is connected to the detectors; the cross-flow exits via the membrane. Sample constituents elute separated according to size and are monitored by the array of detectors.11,12
With AF4/FFF separation, there is no column media to interact with the samples; thus for very high-molar-mass polymers, in comparison to size exclusion (SE)-HPLC, considerably reduced shearing forces emerge. The entire separation is gentle, rapid, and nondestructive without a stationary phase that may interact, degrade, or alter the sample.
Materials and methods
In this study, a stressed VLP sample was analyzed by AF4 using either a standard separation channel (25 cm) or small separation channel (18 cm) (Wyatt Technology Corp., Santa Barbara, CA), both equipped with a 350-µm spacer. An Eclipse2™ separation system (Wyatt Technology Corp.) was used with an Agilent HPLC system (Agilent Technolgies, Palo Alto, CA) equipped with a UV detector and DAWN EOS MALLS detector (Wyatt Technology Corp.). The VLP concentration was determined at 260 nm via UV detection. The MALLS detector was used to determine the average molecular weight of the VLP fractions.
Results
VLP was employed for the development of the AF4 method on an Eclipse2 AF4 system equipped with the standard channel (25 cm). Note that the separation efficiency on the AF4 method can be influenced by manifold parameters such as channel height, membrane material and molecular weight cutoff, eluent composition, focusing time, channel flow and cross-flow profile, and applied drug amount. In preliminary experiments, the following parameters were determined as fixed:
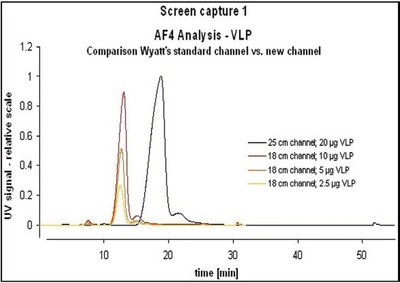
Figure 2 - Comparison of standard channel and shorter channel.
- Spacer—350 µm
- Eluent—20 mM sodium phosphate buffer (pH 7.0) with 150 mM sodium chloride
- Ultrafiltration membrane (regenerated cellulose), molecular weight cutoff—10 kDa
- Channel flow—1.5 mL/min
- Injection flow—0.2 mL/min
- VLP amount—20 µg.
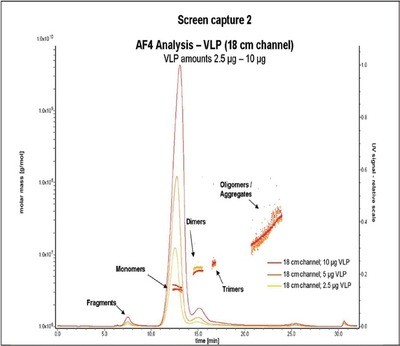
Figure 3 - Comparison of different injection amounts.
Comparative AF4 measurements of VLPs with the standard channel (25 cm) and the shorter channel (18 cm) revealed almost similar peak heights when 20 µg VLP was injected in the standard channel or when 10 µg VLP was injected in the small channel, respectively (Figure 2). Increased peak heights obtained with the new channel are due to sharper peak resolutions. Analysis is therefore possible with significantly less sample (Figure 3). At the same time, analysis time and solvent volume are reduced (Table 1).
Table 1 - Comparison of standard channel and shorter channel
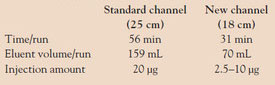
Table 2 - Repeatability of the AF4 analysis of VLP (20 μg/injection)
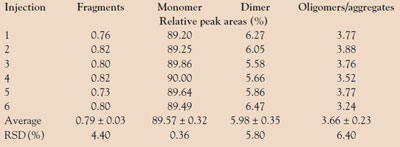
Repeatability
Since AF4 is intended to be used as a reliable tool for the quality control of VLP formulations, repeatability of the method is required. Therefore, a primary focus of the study was to assess the repeatability of the determination of the relative amount of the varying VLP species, expressed as the percentage of total peak areas. The VLP (20 µg/injection) was, according to the instructions of the ICH guideline Q2 (R1), subjected to six independent measurements by AF4. The results, presented in Table 2, demonstrate the repeatability of the applied AF4 method. Even for the VLP fractions present in low amounts (fragments and oligomers/aggregates), a relative standard deviation of less than 7% was determined.
Conclusion
The successful development of AF4 methods for the assessment of VLP compositions was presented. The experiment investigated whether the described AF4 method for VLP, developed for the standard AF4 channel (25 cm), could be optimized by using a new, shorter AF4 separation channel (18 cm). It has been shown that in comparison to the standard channel, the application of the small channel improves resolution, enables shorter equilibration and analysis times, and reduces eluent volumes. At the same time, increased peak heights are obtainable, which in turn lead to lower detection limits, consequently enabling a reduction in the injection amount. Therefore, the new channel technology is a clear improvement for VLP characterization over the standard channel.
References
- Block, S.L.; Nolan, T.; Sattler, C.; Barr, E.; Giacoletti Katherine, E.D.; Marchant, C.D.; Castellsague, X.; Rusche, S.A.; Lukac, S.; Bryan, J.T.; Cavanaugh, P.F., Jr.; Reisinger, K.S. Comparison of the immunogenicity and reactogenicity of a prophylactic quadrivalent human papillomavirus (types 6, 11, 16, and 18) L1 virus-like particle vaccine in male and female adolescents and young adult women. Pediatrics2006, 118(5), 2135–45.
- Ambuehl, P.M.; Tissot, A.C.; Fulurija, A.; Maurer, P.; Nussberger, J.; Sabat, R.; Nief, V.; Schellekens, C.; Sladko, K.; Roubicek, K.; Pfister, T.; Rettenbacher, M.; Volk, H.D.; Wagner, F.; Mueller, P.; Jennings, G.T.; Bachmann, M.F. A vaccine for hypertension based on virus-like particles: preclinical efficacy and phase I safety and immunogenicity. J. Hypertension2007, 25(1), 63–72.
- Maurer, P.; Bachmann, M.F. Therapeutic vaccines for nicotine dependence. Curr. Opin. Molec. Therapeut.2006, 8(1), 11–16.
- www.encyclopedia.com/doc/1G1-185330693.html.
- www.geocities.com.
- Litzen, A.; Walter, J.K.; Krischollek, H.; Wahlund, K.G. Separation and quantitation of monoclonal antibody aggregates by asymmetrical flow field-flow fractionation and comparison to gel permeation chromatography. Anal. Biochem.1993, 212(2), 469–80.
- Moon, M.H.; Giddings, J.C. Size distribution of liposomes by flow field-flow fractionation. J. Pharmaceut. Biomed. Anal.1993, 11(10), 911–20.
- Lee, H.; Williams, S.K.R.; Allison, S.D.; Anchordoquy, T.J. Analysis of self- assembled cationic lipid-DNA gene carrier complexes using flow field-flow fractionation and light scattering. Anal. Chem.2001, 73(4), 837–43.
- Jores, K.; Mehnert, W.; Drechsler, M.; Bunjes, H.; Johann, C.; Maeder, K. Investigations on the structure of solid lipid nanoparticles (SLN) and oil-loaded solid lipid nanoparticles by photon correlation spectroscopy, field-flow fractionation and transmission electron microscopy. J. Controlled Release2004, 95(2), 217–27.
- Thielking, H.; Kulicke, W.M. Determination of the structural parameters of aqueous polymer solutions in the molecular, partially aggregated, and particulate states by means of FFFF/MALLS. J. Microcol. Sep.1998, 10(1), 51–6.
- Schimpf, M. Field Flow Fractionation Handbook; John Wiley & Sons, Inc.: New York, NY, 2000.
- www.wyatt.eu/index.php?id=field_flow_ fractionation.
Dr. Lang is a former Ph.D. student of Prof. Winter, and is currently with Intervet International, GmbH, Untershleissheim, Germany. Dr. Vogt is Head, Process Development and Production, Cytos Biotechnology AG, Schlieren, Switzerland. Mr. Zürcher is Project Manager CMC, Molecular Partners, Zurich, Switzerland, and was previously with Cytos Biotechnology AG. Prof. Dr. Winter is Chair of Pharmaceutical Technology and Biopharmaceutics, Ludwig-Maximilians-University, Butenandtstr. 5, 81377 Munich, Germany; tel.: +49 89 2180 77022; fax: +49 89 2180 77020; e-mail: [email protected].