One need only look around to see that the world is full of surfaces. A great deal of useful chemistry has been developed to give thin films at surfaces desirable properties—coatings and finishes, for example. Nature sometimes creates undesirable thin films, such as the biofilm called plaque on teeth. Many important chemical and biological processes occur in these films either in their production or use. For a solid film exposed to a gas, the gas may adsorb or dissolve in the film, or react catalytically at the surface. In a coating containing volatile components, the components evaporate by absorbing heat, the film mass decreases, and the viscous film becomes a glassy solid. Bacteria will grow on any film containing nutrients. In all of these processes, heat is generated or absorbed, the thin film gains or loses mass, the viscoelastic properties of the solid film change, and the properties desired may be enhanced or destroyed.
The quartz crystal microbalance/heat conduction calorimeter (QCM/HCC) was developed to study these chemical and biological processes in thin films.1–3 Heat conduction calorimetry has been used extensively to measure adsorption energetics in solids, and gas sorption instruments or thermal gravimetric analysis can measure mass release in solids. Rheological measurements of shear and loss modulus are usually done at low frequency on large samples with dynamic mechanical analysis instrumentation. Until the QCM/HCC, however, there has been no instrument that simultaneously measures heat generation, mass uptake or release, and viscoelastic property changes in the same submilligram solid film sample.
QCM/HCC technology
The first commercially available QCM/HCC, the Masscal™ G1 (Masscal Corp., Chatham, MA), employs a patented mass/heat flow sensor4 with a sensitivity sufficient to detect molecular monolayer formation in all signal channels. The mass sensor used in the Masscal G1 is a piezoelectric shear mode resonator made of quartz, termed a quartz crystal microbalance (QCM). When the resonator is electrically driven at its natural acoustical frequency, the decrease in resonant frequency is proportional to the increase in mass per unit area of a thin film deposited at its surface. The thin film may be a polymer, a protein, a paint or coating, a chemical sample, a catalyst, or a metal, but it must adhere to the QCM surface. QCMs have been used for decades in the vacuum deposition industry as monitors of film thickness, and more recently they have found application in electrochemistry (the EQCM).5 The Masscal mass measurement sensor is a 5.00-MHz QCM, 2.54 cm in diam, inexpensive, and easily replaceable. A deposit of 1 μg of sample per cm2 on the QCM surface decreases the resonant frequency by an easily measurable 56.6 Hz. Subsequent mass changes are followed as the film is exposed to atmospheric pressure gas mixtures with varying partial pressures of adsorbing or reacting gases.6
An uncoated QCM has a sharply defined resonance frequency, ν0. The width of the resonance δν is only a few Hz, and the mechanical damping within the quartz that gives rise to this broadening can be determined by measuring an electrical quantity called the “motional resistance” R of the QCM (for typical bare QCMs, R~10 ohms). When thin, stiff films are deposited on the QCM surface, the increase in R is small, but softer, thicker films (i.e., rubbery polymers 5–10 μm thick) can increase R by hundreds or even thousands of ohms. It was shown that the difference in motional resistance of the coated and uncoated QCM is proportional to the shear loss compliance J” of the film.7 For organic films gaining or losing volatile components, changes in J” can be easily measured, and indicate the extent to which the film is being plasticized.
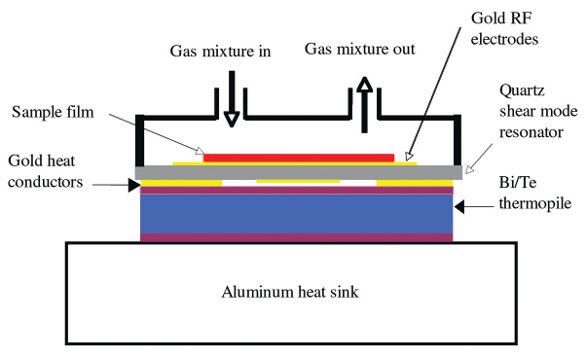
Figure 1 - Cross-sectional view of the mass/heat flow sensor and sample chamber of the QCM/HCC. In the laboratory, a thin film sample (red) is coated on the top gold electrode of the quartz shear mode resonator (QCM). The QCM is then inserted between the thermopile and the sample chamber. The experiment consists of varying the composition of the gas mixture at constant temperature and observing changes in the resonant frequency and motional resistance of the QCM and the thermal power flowing between the QCM and the aluminum heat sink via the thermopile.
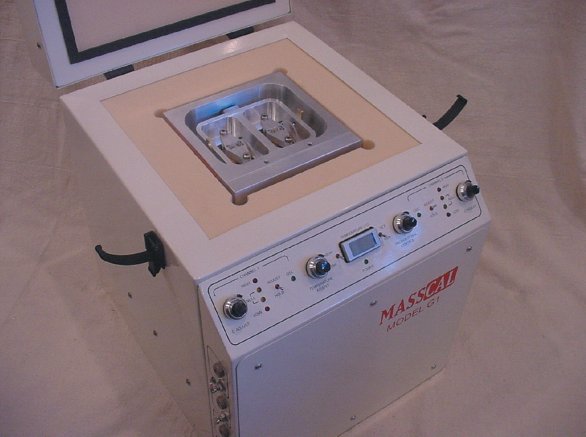
Figure 2 - Top view of the Masscal G1 showing the sample chamber inside two layers of aluminum thermal shields, further insulated with several inches of high-quality polyurethane foam.
In the Masscal mass/heat flow sensor (Figure 1), the QCM is thermally coupled to a heat sink through a Peltier thermocouple plate. Any heat flow generated by processes in the thin film on the QCM’s upper surface is detected as a voltage change by the thermocouple plate—the heat conduction calorimetry (HCC) principle. In the Masscal G1 (Figure 2), the mass/heat flow sensor is placed in a quasi-adiabatic thermal environment (temperature controlled to ±0.01 °C without using a circulating bath) and is exposed to a slow flow of gas mixture at ambient pressure. Three quantities are measured simultaneously: 1) the thermal power P(t) (to 0.5 μW), 2) the mass change m(t) (to 2 ng/cm2), and 3) the change in motional resistance R(t) (to ±0.1 ohm) of the damped oscillator when the sample film takes up, releases, or reacts with the probe gas. Any program of gas composition versus time is achievable through mass flow controllers. A series of stepwise changes in the concentration of the volatile component is the standard mode.6
Any solid that can be prepared as a thin film on a gold-coated quartz substrate is amenable to study. Films of 1–2 cm2 area and 0.1–10 μm thickness are optimal. Methods of film preparation thus far have included spin-coating, spray-coating, and electrochemical deposition. Solids studied have included Pd and Pt metal films, an aliphatic polyurethane polymer, the proteins lysozyme and myoglobin, the molecular solids C60 and C60/piperazine, a polymeric mesoporous thin film, pharmaceutical film-coat materials, and nutrient-containing agar films.
Applications
The QCM/HCC has many applications because it is a new instrumentation concept in thermal analysis and calorimetry, applicable to thin films. Examples of applications already proved feasible or in progress are given in Table 1.
Table 1 - QCM/HCC applications
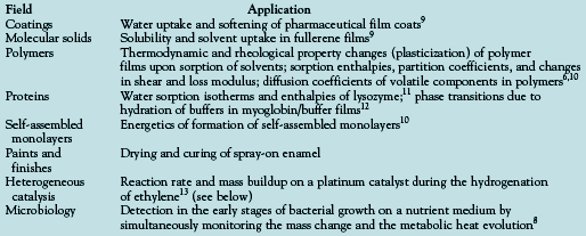
In 2003, Masscal Corp. contracted with E.I. DuPont Co. (Wilmington, DE) to study the feasibility of measuring binding enthalpies for certain materials in solution to thin films deposited on the surface of a QCM. These measurements showed that it is possible to perform QCM/HCC measurements of reactions at thin film surfaces with solution-based reagents, but this capability is not yet ready for commercialization. Experiments conducted at the author’s laboratory at Drexel University (Philadelphia, PA) in 2004 show that it is possible to detect protein–ligand binding with all three measurement channels in a thin film of human serum albumen exposed to solutions of the organic ligand warfarin.8
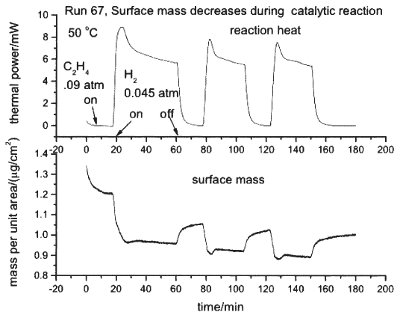
Figure 3 - Thermal power and mass change during the catalytic reaction of hydrogen and ethylene at a platinum surface. The time scale is in minutes. When only ethylene is present in the nitrogen carrier gas stream, no heat is generated at the catalyst surface. When hydrogen is added, there is a large heat signal due to the exothermic reaction of hydrogen and ethylene at the catalyst surface. The lower panel shows that the mass of the adsorbed film on the platinum catalyst decreases when the reaction occurs.
In mid-2003, Masscal Corp. received a Phase I SBIR Grant from the U.S. Department of Energy to determine the feasibility of developing a real-time monitor of catalytic surfaces for petrochemical synthesis using a mass/heat flow sensor. Measurements taken under this contract show that it is possible to employ the QCM/HCC to monitor the rate of the reaction H2 + C2H4 → C2H6 at a platinum catalyst surface on the QCM by detecting the heat evolved, while at the same time measuring the change in mass of the adsorbed surface layer.13 An example of the data is shown in Figure 3. When only ethylene is flowing across the platinum surface, no heat is evolved. As soon as hydrogen is added, however, the reaction proceeds and heat is evolved. Note, though, that the surface mass decreases as soon as the hydrogen is added, and then increases when the hydrogen is turned off. This is believed to be due to the reaction of hydrogen with an adsorbed surface layer of adsorbed ethylenic intermediate species. The QCM/HCC is the only device that simultaneously detects heat evolution and mass changes on the same thin-film catalyst.
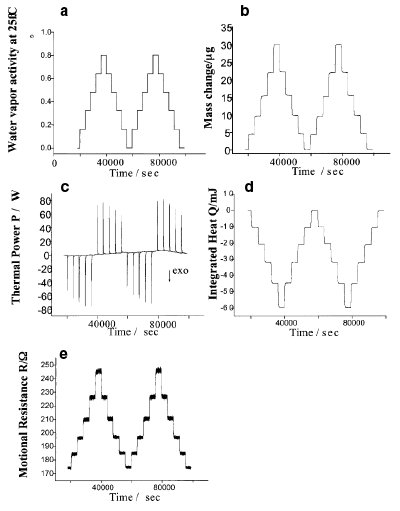
Figure 4 - Data collected when a film of the polyurethane Tecoflex 8.5 μm thick absorbs water vapor. The time scale is in seconds. a) Program of water vapor partial pressure or relative humidity flowing through the sample chamber. b) Mass of water vapor absorbed and desorbed during the two full cycles. c) Exothermic heat flow pulses observed every time water vapor is absorbed, followed by the endothermic pulses when the water evaporates. d) Time integral of (c) and heat changes in milliJoules for each absorption/desorption event. The ratio of the step changes in (d) and (b) yield the sorption enthalpy of water vapor in Tecoflex, and the mass changes in (b) give the water sorption isotherm. e) Changes in motional resistance, or the damping of the QCM resonator, caused by water vapor sorption in the polymer film. As the film absorbs moisture, it is plasticized and the damping increases.
Figure 4 shows typical data for water vapor sorption by an aliphatic polyurethane used in medical applications, with the trade name Tecoflex™6 (Noveon Thermedics Polymer Products, Wilmington, MA). The film thickness was 8.5 μm. Figure 4a shows the applied water vapor activity, programmed through the control software and generated with mass flow controllers. Figure 4b is the resulting mass change due to the absorption and desorption of water in the Tecoflex film. Figure 4c is the corresponding thermal power signal. Notice that every time the relative humidity increases, there is an exothermic heat pulse coming from the water vapor absorbing in the film and releasing heat in the process. Figure 4d is the time integral of the previous trace, the “integrated heat.” Figure 4e is the motional resistance change of the film.
The data in Figure 4 are sufficient to determine both the enthalpy of water vapor sorption and the water vapor sorption isotherm in Tecoflex (mass of water vapor per unit mass of polymer film as a function of relative humidity). The motional resistance trace can be interpreted in terms of the plasticizing effect that water or any other small organic solvent has when dissolving in a polymer film. The film is “plasticized” by the water vapor and becomes softer. In terms of the viscoelastic properties of polymers, it can be shown that the change in motional resistance between the coated and uncoated film is proportional to the film’s loss compliance. Thus, Figure 4e shows that the loss compliance of Tecoflex increases as it absorbs moisture.
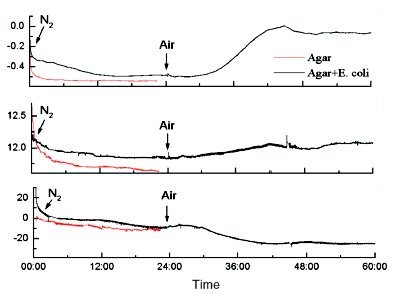
Figure 5 - Detection of viable bacteria using the QCM/HCC. The time scale is in hours. E. coli were inoculated on a thin film of agar growth medium and exposed to nitrogen for the first day. Since anaerobic growth of E. coli is slow, no signal changes due to microbial growth are evident. When air is admitted, the thermal power increases in the first few hours, followed by later increases in both surface mass and motional resistance. These signals show that viable E. coli growing on the surface of the agar-coated QCM can easily be detected.
Figure 5 shows the signals from a nutrient film of agar placed on the QCM and then inoculated with E. coli. On the first day of the experiment, the atmosphere was nitrogen, and no growth was observed. When the ambient atmosphere was changed to air, the growth of E. coli was observed as the evolution of heat, the increase in mass, and the increase in motional resistance.8
According to a market report,14 technological developments in the $20 billion U.S. analytical instrument industry are critically dependent on the development of new-generation instruments. The author contends that the QCM/HCC is one such instrument. Below are the report’s criteria for new-generation instruments, and the way in which the QCM/HCC meets these criteria:
- “It must have some feature that performs at least an order of magnitude better than a preceding model.” This feature is the quartz crystal microbalance, whose sensitivity to mass measurements (±2 ng) far exceeds analytical mechanical microbalances on the market.
- “The design with regard to the combination of components has to be new.” No one has previously combined a quartz crystal microbalance with a heat flow sensor. The resulting mass/heat flow sensor is covered by an issued patent with more than 50 claims (licensed to Masscal Corp.).
- “The instrument should have performance capabilities not seen in previous models, and should be the first on the market for a given application.” The QCM/HCC can measure change in mass, heat flow, and viscoelastic damping in a thin film exposed to a gas. The Masscal G1 is the first such instrument on the market.
Several other potential applications of the QCM/HCC include:
- Characterizing the thermodynamic stability and resistance to oxidation of the supramolecular nanoscale structures of nanotechnology.
- Determining optimum moisture content in protein pharmaceutical formulations.
- Testing polymers as gas sensors.
References
- Smith AL, Shirazi H, Wadso I. The QCM/HCC: simultaneous, isothermal, high sensitivity measurements of mass change and heat flow in polymer and fullerene films. In: Ruoff RS, Kadish KD, eds. Recent advances in the chemistry and physics of fullerenes and related materials, vol. 98–8:576–85. San Diego, CA: Electrochemical Society, 1998.
- Smith AL, Shirazi HM. Quartz microbalance microcalorimetry: a new method for studying polymersolvent thermodynamics. J Therm Anal Calorim 2000; 59:171–86.
- Smith AL, Mulligan SR, Tian J, Shirazi H, Riggs JC. A mass/heat flow sensor combining shear mode resonators with thermoelectrics: principles and applications. Presented at IEEE Frequency Control Symposium, Tampa, FL, May 2003.
- Smith AL. Mass and heat flow measurement sensor. 2000. U.S. patent no. 6,106,149.
- Buttry DA, Ward MD. Measurement of interfacial processes at electrode surfaces with the electrochemical quartz crystal microbalance. Chem Rev 1992; 92:1355–79.
- Smith AL, Mulligan SR, Shirazi HM. Determining the effects of vapor sorption in polymers using the quartz crystal microbalance/heat conduction calorimeter. J Polym Sci Part B Polym Phys 2004; 42:3893–3906.
- Smith AL. Rheological measurements in thin films plasticized by solvents: an application of the quartz crystal microbalance. J Polym Sci Part B Polym Phys 2004, in press.
- Smith AL, Zilberman G. Detection of vital bacteria and protein ligand binding using the QCM/HCC. In: Rich M, ed. 2004 NATAS. Williamsburg, VA: NATAS, 2004.
- Tian J. Applications of quartz crystal microbalance/heat conduction calorimetry. Ph.D. thesis, Drexel University, Philadelphia, PA, 2003.
- Shirazi HM. Quartz crystal microbalance/heat conduction calorimetry (QCM/HCC), a new technology capable of isothermal, high sensitivity, mass and heat flow measurements at a solid/gas interface. Ph.D. thesis, Drexel University, Philadelphia, PA, 2000.
- Smith AL, Shirazi HM, Mulligan SR. Water sorption isotherms and enthalpies of water sorption by lysozyme using the quartz crystal microbalance/heat conduction calorimeter. Biochim et Biophys Acta (BBA)–Protein Structure and Molecular Enzymology 2002; 1594:150–9.
- Mulligan SR. The QCM/HCC and applications in studying the thermodynamic and rheological properties of polymer and protein thin films at a gas/solid interface. Ph.D. thesis, Drexel University, Philadelphia, PA, 2003.
- Smith AL, Smith FC, Shirazi HM. Using quartz crystal microbalance/heat conduction calorimetry to monitor heterogeneously catalyzed reaction rates and changes of surface mass: hydrogenation of C2H4 on palladium and platinum. In: Rich M, ed. 2004 NATAS. Williamsburg, VA: NATAS, 2004.
- Wrotnowski C. The new generation of analytical instruments. Norwalk, CT: Business Communications Corp., 1998:200.
Dr. Smith is President, Masscal Corp., 96 A. Leonard Way, Chatham, MA 02633, U.S.A.; tel.: 508-241-8628; fax: 508-348-0303; e-mail: [email protected].