Environmental samples, including water and soils, extracted with an organic solvent must typically be dried and concentrated before analysis. Experience has shown that the drying and concentration steps can result in significant recovery losses of analytes. These losses are due to several factors. One, it is known that sodium sulfate can adsorb compounds or allow excess residual water to pass through, which could impact the final quantitation. Two, the concentration step can cause a loss of the more volatile compounds, due to their higher vapor pressures. When these two factors are combined, the total impact can be a significant drop in recovery values.
A concentrator system has been designed specifically to eliminate these and other problems. Using a unique combination of an in-line hydrophobic membrane solvent dryer, independent internal heaters, vacuum, and optimized gas sparge system, the DryVap™ concentrator system (Horizon Technology, Inc., Atkinson, NH) is able to achieve high recoveries at a fast evaporation rate, even on volatile compounds. One reason high recovery values are possible is because of an internal thermocouple. When the solvent level reaches a point just above the heater, the thermocouple senses this, and turns off the heater power. The evaporation tubes remain under vacuum, and the gas sparge continues a gentle evaporation process until the solvent level reaches the end point sensor. At this point, the evaporation process is complete. It is important to note that it is during the final stage of evaporation, when no heat is being applied, that the tube nipple becomes cool. Maintaining a cool environment is critical to achieving high recoveries of the more volatile compounds.
The following comparison of the DryVap system to the traditional Kuderna Danish (KD) method will review several classes of compounds for recovery values versus the total time required.
Instrumentation and method conditions
The instrumentation used included the DryVap dryer concentrator system, the Hewlett Packard GC 5890 and MS 5971, and KD tube apparatus.
Method conditions were as follows:
- Twenty-five milliliters of n-hexane was used for all concentration runs.
- Runs were made using gasoline, aliphatic hydrocarbons, and chlorinated pesticides.
- Main vacuum was set to 15 in. of Hg.
- Nitrogen gas pressure was set to 15 psi.
- Heater power was set between 1.0 and 4.8 V (max.).
- Final analysis was by GC-MS.
Benchmark test
Nitrogen blow-down method
In order to establish a benchmark comparison, 25 mL of hexane was placed into a 25-mL KD concentrator tube and spiked with 20 μg of the aliphatic hydrocarbon mix, C8–C21. Aliphatic hydrocarbons were chosen because of their nonpolar nature and low intermolecular forces, which makes them more difficult to concentrate without inducing a loss. The KD tube was placed into a water bath (45 °C), and a gentle stream of nitrogen was blown down onto the solvent surface. The gas inlet needle was constantly adjusted to maintain an equal distance between the needle and the solvent surface. The solvent volume was taken down to a volume of 0.8 mL.
Table 1 - Comparison of recovery values of KD tube and DryVap system unit for aliphatic hydrocarbons
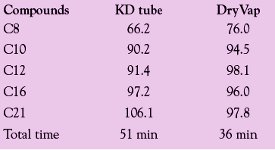
The results, shown in Table 1, were used as the benchmark. Note the total concentration time of 51 min.
DryVap method
The same mix was then placed into the 200-mL DryVap concentrator tube and placed onto the DryVap system. The heater power setting was set to 1.0 V. The solvent was concentrated down to the automatic end point of 0.8 mL. Note that the recoveries between both techniques are very similar, but the DryVap system concentrated the solvent down in less time.
Based on the above data, which show that the DryVap system is able to concentrate the aliphatic hydrocarbons down in considerably less time than a standard nitrogen blow-down technique and provide comparable recoveries, the next set of tests were conducted.
Table 2 - Average recoveries for gasoline
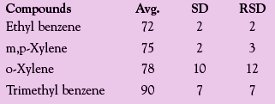
Further results
The next series of concentration tests were performed using gasoline. Gasoline was chosen for its overall volatility. Twenty-five milliliters of hexane was spiked with 25 μL of gasoline and concentrated. The heater power setting used for the gasoline sample was 3.5 V. Three runs were made and the results averaged. Total run times were approx. 12 min to concentrate from 25 mL to 0.8 mL. Table 2 shows the results of this test.
These results indicate that the DryVap system was able to remove the hexane solvent and still maintain good recoveries for these four gasoline components.
Table 3 - Average recoveries using the DryVap concentrator for chlorinated pesticides from Method 508.1
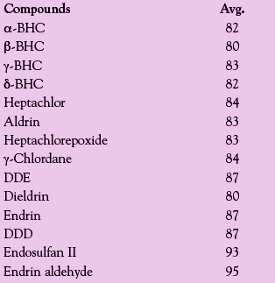
The next series of tests employed chlorinated pesticides. Forty micrograms of chlorinated pesticides was added to 25 mL of hexane, and the samples were concentrated down to 0.8 mL. A power setting of 3.0 V was used. Three runs were made and the averaged results are shown in Table 3. The data indicate good recoveries for these compounds, given that the average evaporation times were approx. 10–12 min.
Optimization parameters
Reducing the time required to evaporate a sample without negatively impacting the recovery values isthe desire of most laboratories. The data in Table 1 showed comparable recoveries of the DryVap to the nitrogen blow-down technique, but with a significant reduction in time. The next step was to determine how fast the evaporation process could be driven, and the associated impact on recoveries. Therefore, a series of runs were made using the aliphatic hydrocarbons. Again, these compounds were chosen because C8 and C10, due to their nonpolar nature and high vapor pressure, are difficult to concentrate from hexane without inducing loss. Five runs were made with the heater power voltage at 1.0, 2.0, 3.0, 4.0, and 4.8 (max.) V, respectively. Figure 1 shows that higher heater power settings had no significant negative impact on recovery values.
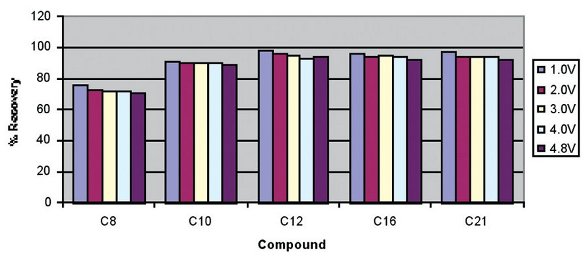
Figure 1 - Impact of heater voltage power on the recovery of aliphatic hydrocarbons.
When the thermocouple senses the solvent level, the heater is turned off, leaving only the vacuum and the gas sparge on. By using a higher power voltage, the total time required to concentrate the sample from 25 to 0.8 mL was reduced significantly. Figure 2 shows the evaporation rates at various heater power settings. The blue column (the left columns depicted at each power setting) shows the evaporation rate when the heater is on, while the purple column (middle column at each power setting) shows the evaporation rate when the heater is off and only the vacuum and gas sparge are on. The white column (right column) is the combined evaporation rate at various heater power settings with heat on and off. As would be expected, faster rates can be achieved with higher heater settings.
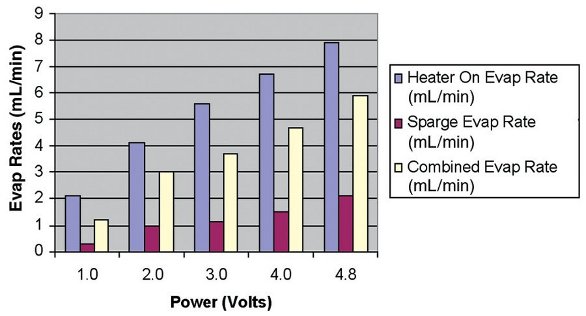
Figure 2 - Evaporation rates of hexane as a function of heater voltage power.
By comparing the evaporation rates of Figure 2 with the recovery values of Figure 1, it can be seen that while higher heater power settings can increase the evaporation rates, there is apparently little adverse impact on the recoveries. It is interesting to note that with the higher heater power settings, the evaporation rates during the gas sparge cycle are also greater. This is due to the latent heat that is retained by the glass evaporation tube. It is speculated that because the evaporation tube is completely sealed during the concentration process, at higher power settings the condensed solvent collects on the internal surfaces and is responsible for retaining the volatile fraction. In addition, once the heater is turned off, the evaporation tube and glass nipple remain at a much cooler temperature. This cooler temperature is critical to achieving better recoveries of the more volatile compounds.
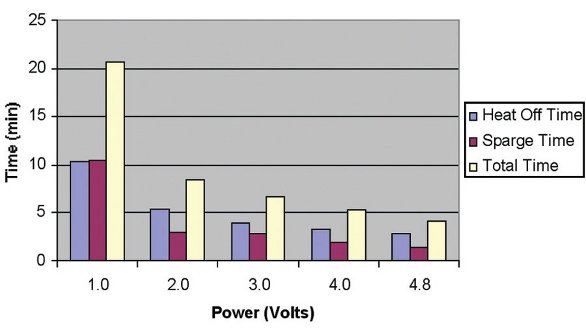
Figure 3 - Total evaporation times to evaporate 25 mL hexane to 0.8 mL.
For all of the above runs, the total time to evaporate the solvent, both with heat on and heat off, was recorded. Figure 3 shows the evaporation times from 25 to 0.8 mL as a function of the heater power setting. The blue column (left column) is the time required to go from 25 mL solvent until the heater is turned off, while the purple column (middle column) is the time required during the gas sparge stage. The white column (right column) shows the total time required to evaporate from 25 to 0.8 mL. As can be seen, at a power setting of 4.8 V (max.), the total time was less than 5 min.
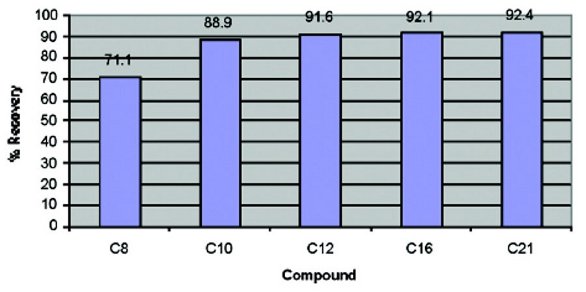
Figure 4 - Recovery values of C8–C21 at heater power 4.8 V.
In order to determine the impact that vacuum might have on the recoveries, another run was made with a vacuum setting of 10 in. of Hg. A heater power setting of 4.8 V (max.) was used. Figure 4 shows the recovery values of C8–C21 at this reduced vacuum setting. The recovery values indicate that using a lower vacuum increased the recoveries of the compounds, as compared to Figure 1. Therefore, lower vacuum levels are acceptable since the total evaporation time only increased to just less than 8 min.
Conclusion
The DryVap system enables rapid and fully automated concentration of solvent extracts without inducing a loss in the more volatile or nonpolar compounds. The combination of an internal heater, vacuum, gas sparge, and automatic heater shutoff, while continuing with the gentle gas sparge, provides the proper conditions to remove the solvent while minimizing compound loss. The concentrator system combines what were once multiple manual steps into one system with excellent comparison data to the traditional KD method.
Mr. Johnson is Chief Technical Officer, Horizon Technology, Inc., 8 Commerce Dr., Atkinson, NH 03811, U.S.A.; tel.: 603-893-3663; fax: 603-893-4994; e-mail: [email protected].