One-third of the world’s population is infected with tuberculosis, and one person dies every 15 sec from the disease. The existing treatment of tuberculosis takes nine months and relies on drugs developed before the 1970s. There is an urgent need to develop new drugs to reduce treatment time and to combat the emergence of multidrug-resistant strains of tuberculosis. One of the goals of the Novartis Institute for Tropical Diseases (Singapore, Malaysia) is to develop new drugs for the treatment of tuberculosis (TB), particularly multidrug-resistant (MDR) TB, and shorten the current treatment times from 6–9 months to 2 months.
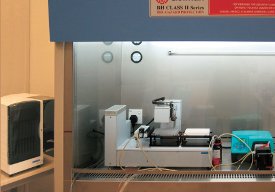
Figure 1 - Nanodrop II stage module inside a Biosafety Level II containment cabinet. (Photograph courtesy of Novartis Corp., Basel, Switzerland.)
The Institute’s current approach is to develop a relatively low-volume, simple, robust, and reproducible assay methodology to test potential antibiotics against proliferating Bacillus Calmette-Guérin mycobacteria (the strain currently used in vaccinations against Mycobacterium tuberculosis). There are several key challenges in setting up the laboratory to perform such assays reliably, including the need for transfers to occur in sterile, biologically isolated conditions (e.g., within a Biosafety Level II containment cabinet), and the precise and reproducible transfer of stock compounds into the assay plates without cross-contamination. Furthermore, the isolation of the compound transfer device in the containment cabinet necessitated some means for it to perform tip self-cleaning and to operate for relatively long periods of time without maintenance or loss of precision. The accurate dispensing of low amounts of compound was also seen as desirable, allowing for both conservation of test compounds and the ability to miniaturize the assay.
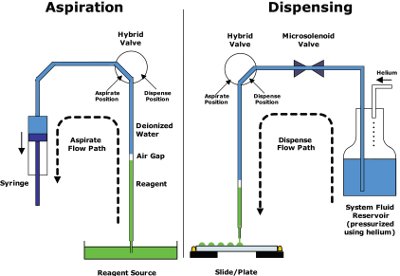
Figure 2 - The fluidics architecture of the Nanodrop isolates the sample from the moving parts (such as valves and syringes) in the flow path, which only encounter deionized water. Reagent is aspirated by syringe action and is dispensed by pulsing pressurized fluid through a microsolenoid valve. The design enables precise control of dispense volumes (due to the use of microsolenoid valves), reliability (due to sample isolation from the flow path), and (combined with tip wash routines) virtual elimination of cross-contamination. (Illustration courtesy of Innovadyne Technologies, Inc.)
For the stock compound transfer portion of the assay, a Nanodrop™ II (Innovadyne Technologies, Inc., Santa Rosa, CA) was evaluated. The small footprint of the stage, and the separation of the stage and fluidics modules in the design, make possible the inclusion of the stage within the containment cabinet, as shown in Figure 1. Additionally, the aspirate–dispense architecture of the device (noncontact, with valves isolated from the flow path) (see Figure 2) with customizable tip washing procedures showed promise for meeting the Institute’s requirements. The next step was to validate the performance of the system in the areas of dispensing precision, accuracy, and cross-contamination suppression.
Validation of stock transfer performance
The performance of the system was validated for transferring stock test compound in 90% v/v dimethyl sulfoxide (DMSO)/water using the dye Eosin Yellow, which was dissolved in the DMSO solution at a concentration of 100 mM. Accuracy and precision were assessed by extrapolating the volume of dye dispensed using the Nanodrop II from an Eosin Yellow calibration curve, with volumes of 250, 500, and 1000 nL dispensed in three 96-well plates per volume. The average precision (% CV) was 6.19, 2.76, and 3.16 for 250, 500, and 1000 nL volumes, respectively (see Figure 3) and the accuracy for all volumes showed a deviation from expected volume transferred of less than 10%.
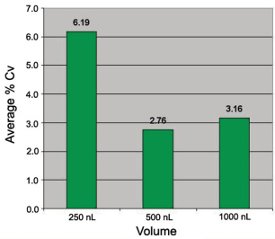
Figure 3 - To validate the Nanodrop’s performance, the average dispensing precision (whole-plate CV) was measured of three volumes of a stock test compound (Eosin Yellow dye in 90% DMSO/water) transferred into three 96-well plates for each volume. The results were well within the published specifications for the instrument.
Prevention of test compound cross-contamination is generally a concern when using fixed tips to transfer test compounds. Several tip wash routines were evaluated for their capacity to eliminate sample carryover on the Nanodrop II. Figure 4 shows the results for two wash procedures. The first bar of each graph refers to control data for cells only (0% inhibition of cell growth). The second bar of each graph includes cells plus test compound (with nM IC50) at 20 μM (100% inhibition of cell growth). The third bar shows the efficiency of washing by running the control again after the wash procedure under study. The first wash procedure, including only a wash with system liquid after each column of compounds was transferred, resulted in 26.4% inhibition of cell growth, indicating compound carryover on the tips. The second procedure using three washes with 90% v/v DMSO/water (from a recirculating reservoir) and then a wash with system liquid (deionized degassed water), after each column of compounds was transferred, resulted in no significant inhibition of cell growth, indicating no compound carryover on the tips.
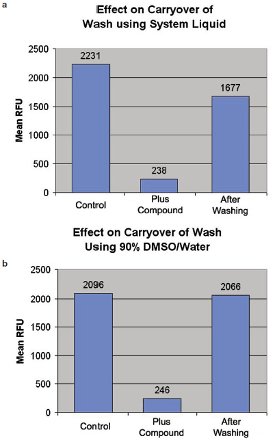
Figure 4 - Two separate wash routines were evaluated for their effect on carryover contamination of a test compound with strong cell growth inhibition. In both cases, a control assay was run in which the relative number of cells was measured in relative fluorescence units (RFUs) followed by the fluorescence after the test compound was added. Following the addition of this test compound, the tips and dispense tubing were washed either with a rapid flow of system fluid from the Nanodrop fluidics, or by aspirating and dispensing 90% DMSO three times into the tips and dispense tubing. The single system fluid wash resulted in significant (26.4%) inhibition of cell growth, whereas the three DMSO washes resulted in negligible growth inhibition.
The Bacillus Calmette-Guérin mycobacteria proliferation assay was based on the methodology of Collins and Franzblau,1 with the exception that the current study was performed at a final volume of 50 μL (rather than the final volume of 200 μL used by Collins and Franzblau). The assay was validated by screening a diverse set of compounds (a total of 8800 compounds in 100 96-well plates) from the Novartis compound archive at a single concentration of 20 μM. A volume of 500 nL of 2 mM test compound in 90% v/v DMSO/water was transferred to sterile, clear, ½-well 96-well plates (Corning Costar Corp., Acton, MA) using the Nanodrop. Fifty microliters of Bacillus Calmette-Guérin mycobacterium (diluted to an A650 nm of 0.02 from a flask culture) was added to the plates using a WellMate (Matrix Technologies Corp., Hudson, NH), and the cells were incubated for four days at 37 °C and 100% humidity. Twelve microliters of 1:1 Alamar Blue (Serotec, Raleigh, NC):Tween 20 was added to the plates using a WellMate, and the cells were incubated for 24 hr at 37 °C and 100% humidity. The resulting fluorescence was detected using an excitation wavelength of 540 nm and an emission wavelength of 590 nm using a Safire2 (Tecan Group Ltd., Männedorf, Switzerland).
The screen was run in six batches (one batch per week) consisting of 10–25 plates, with a control plate included in each batch. The screen was run over an extended period to determine if the assay was stable over time. Control plates included a streptomycin dose-response curve (11 concentrations from highest concentration of 20 μM diluted serially twofold in 90% DMSO/water). The minimum inhibitory concentration (equivalent to IC90) for streptomycin from all control plates was 0.0912 ± 0.0093 μM (mean ± standard error of the mean). Data from the test set of compounds were analyzed as a whole, and the histogram in Figure 5 shows the distribution of % inhibition.
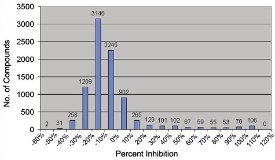
Figure 5 - To validate the assay, a total of 8800 compounds were tested for their percentage of growth inhibition of Bacillus Calmette-Guérin mycobacteria. Each bar represents the number of compounds within a similar percent inhibition category. While the vast majority had near-zero inhibition, several compounds (toward the right edge of the histogram) have been identified as potential antibiotic candidates for further study.
The signal-to-background ratios were 7.8, 8.2, 10.5, 9.4, 12.3, and 12.6, and the z-factors were 0.91, 0.80, 0.92, 0.88, 0.91, and 0.89 for the six runs. The average z-factor for all 100 plates tested was 0.89, as shown in Figure 6. (Note: z-factor is a standard metric used to determine the validity of the results data, as described in Ref. 2. A value of 1 is perfect, 0.5–1 indicates an excellent assay, 0–0.5 indicates a marginal assay, and less than zero indicates that the assay is essentially useless.)
Using a cut-off response of the mean % inhibition + 3 standard deviations from the mean, 270 compounds were selected for reconfirmation by performing dose-response curves (eight concentrations from the highest concentration of 20 μM diluted serially twofold in 90% DMSO/water). Again, the Nanodrop was used to transfer the serially diluted compounds to assay plates, and a reconfirmation rate (i.e., compounds giving curves with an inhibition of a least 50% at the highest compound concentration) of 80% was achieved using this methodology. The quality of the screen was further confirmed by the identification of the antibiotic, rifampicin, known to be active against Mycobacterium tuberculosis, within the compound library. Several novel compound classes were identified from this screen and are being followed up as potentially interesting antibiotics.
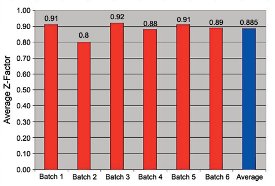
Figure 6 - The assay quality of each plate was evaluated using a z-factor.2 The histogram shows high data quality that is consistent over the total sample size of 100 plates.
The Nanodrop simplified the assay by allowing the direct pipetting of test compounds into each assay plate with high accuracy and precision, permitting conservation of compound usage and leading to a highly reproducible assay. Positioning of the device in a biosafety cabinet has enabled the sterility of the assay plates to be preserved. As of this publication, more than 500 plates have been screened using the assay with no loss in the performance of the assay. Future work includes moving the assay to 384-well plates, utilizing the same assay format with Mycobacterium tuberculosis, and further miniaturization.
References
- Collins, L.S.; Franzblau, S.G. Microplate Alamar Blue assay versus BACTEC 460 system for high throughput screening of compounds against Mycobacterium tuberculosis and Mycobacterium avium. Antimicrob. Agents Chemother. 1997, 41, 1004–9.
- Zhang, J.H.; Chung, T.D.; Oldenburg, K.R. A simple statistical parameter for use in evaluation and validation of high throughput screening assays. J. Biomol. Screen. 1999, 4(2), 67–73.
Additional reading
Dye, C. Global epidemiology of tuberculosis. Lancet2006, 367, 938–40.
Dye, C.; Watt, C.J.; Bleed, D.M.; Hosseini, S.M.; Raviglione, M.C. Evolution of tuberculosis control and prospects for reducing tuberculosis incidence, prevalence, and deaths globally. JAMA2005, 293, 2767–75.
Kremer, L.S.; Besra, G.S. Current status and future development of antitubercular chemotherapy. Expert. Opin. Investig. Drugs2002, 11, 1033–49.
Nunn, P.; Williams, B.; Floyd, K.; Dye, C.; Elzinga, G.; Raviglione, M. Tuberculosis control in the era of HIV. Nat. Rev. Immunol. 2005, 5, 819–26.
Doktycz, M.J.; Johnson, J.E.; Cornett, M.J. Hybrid valve structure for high-throughput, low volume liquid handling applications. JALA Oct 2004, 9(5), 250–6.
The authors are with the Novartis Institute for Tropical Diseases, 10 Biopolis Rd., #05-01 Chromos, Singapore 138670, Malaysia; tel.: +65 67222900; fax: +65 67222910; e-mail: contact.[email protected].