The first calorimetric test most of us performed was probably either the classic Styrofoam cup test or the more sophisticated bomb (combustion chamber) calorimeter, which allowed the measurement of heat changes on large samples with remarkable accuracy. In fact, the bomb calorimeter was a standard apparatus used in scientific and industrial laboratories for many years.
Ironically, today’s ultrasensitive calorimetry is instrumental in analyzing and developing pharmaceutical and polymer products, and saving researchers, chemists, and engineers such extensive time and money that it might seem one of the best-kept secrets in product development today.
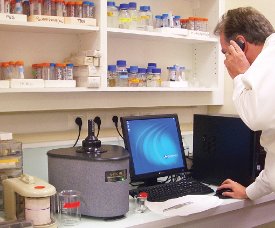
Figure 1 - Today’s ultrasensitive calorimeters provide a unique approach for analyzing molecular structures such as proteins, nucleic acids, and their complexes.
Modern calorimetry has transformed and advanced to such a degree that today its acute sensitivity and flexibility have made it an indispensable tool throughout the life sciences. Ultrasensitive calorimetry now plays an integral role in biotechnology, providing researchers with essential information on the thermodynamics, structure, stability, and functionality of proteins, nucleic acids, lipids, and other biomolecules (Figure 1). Ultrasensitive calorimetry is a vital tool for R&D in pharmaceuticals, genetics, energy, materials—in almost any area where the measurement and controlled manipulation of substances and interactions is required at the molecular level.
Ultrasensitive calorimeters enable researchers and product developers to obtain thermodynamic information that was previously difficult or even impossible to gain with earlier models or by other techniques. The capabilities of advanced, ultrasensitive calorimeters are enormously helpful because of the amount and accuracy of the data they extract, as well as their abilities to obtain these data using very small samples.
One advantage of calorimetry is that it eliminates the need to immobilize, add chromophores, or make any modifications to ligands or macromolecules in order to test materials. The user simply places the material in solution exactly as it would occur in its normal state.
Conversely, using techniques such as spectroscopy requires researchers to use some sort of chromophore, something that can be detected in order to follow a reaction. When such modifications are made to a biopolymer or the environment in which it is being tested, the results may not be the same as would occur in a normal state.
It is highly advantageous to put a biopolymer into solution so it is “folded up” in the natural state, instead of being chemically modified, and in an environment that is similar to the environment of interest. Because an ultrasensitive calorimeter enables such a technique, more reliable information is extracted from the test.
Sensitivity is a by-product of sample size, signal-to-noise, and baseline stability. Today’s calorimeters incorporate new control capabilities that produce baseline stability that previously has not been available. This control also makes the instrument less susceptible to external environmental influences, and with the pressure control capabilities built into the newer models, the instruments are now appropriate for the study of volumetric properties (pressure perturbation) of biopolymers.
A good example of the usefulness of ultrasensitive calorimetry in biotechnology is in the development of new pharmaceuticals. Because many new drugs are proteins, they have stability issues. Even if a drug existed that could cure everything, if it was thermally unstable, it could have a shelf life that would render it useless. Therefore, obtaining the best data possible showing the stability of the compound would be very important. With that information, one can optimize that stability and achieve the necessary shelf life. Today’s calorimetric instruments make this possible.
Two of the most popular and reliable ultrasensitive calorimetric techniques are differential scanning calorimetry (DSC) and isothermal titration calorimetry (ITC). Both principles are designed and optimized for analysis of biomolecules in solution, and are widely used in academic, research, and industrial laboratories throughout the world.
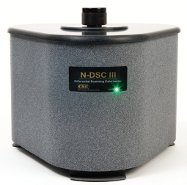
Figure 2 - The Nano DSC series III (Calorimetry Sciences Corp., Lindon, UT) is a highly sensitive calorimeter for researchers interested in studying biopolymer stability and structure.
DSC (Figure 2) measures heat as a function of changing temperature. It is typically used to discern a wide range of thermal transitions in biological systems and the thermodynamic parameters associated with these changes.
ITC (Figure 3) is typically used for monitoring a chemical reaction initiated by the addition of a binding component, and has become the method of choice for characterizing biomolecular interactions.
Traditionally, DSC has been used for stability. This is especially useful to the pharmaceutical industry in the design of advanced drugs. Modifications can be made to a compound or its buffer, and then it can be determined whether the modification stabilized or destabilized the compound.
ITC is the most popular type of ultrasensitive calorimetry today. In the pharmaceuticals area, this technique is used in the testing of drugs that target a very specific area in the human body, possibly a particular gene or receptor. One of the most common uses of ITC is to monitor interactions. It enables the user to measure the energy of those interactions and how tightly a ligand will bind to a gene or receptor. Various biomolecular interactions can be studied using ITC, such as protein–ligand, protein–protein, protein–DNA, antibody–antigen, and so forth.
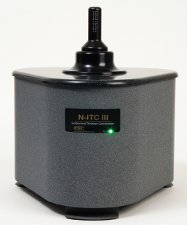
Figure 3 - The Nano ITC series III is designed specifically for the study of biopolymer ligand interactions.
While ITC is particularly suitable to follow the energetics of an association reaction between biomolecules, the combination of ITC and DSC provides a more comprehensive description of the thermodynamics of an associating system.
Calorimetry Sciences Corp. (CSC) manufactures a variety of instrumentation and supporting software, including nanowatt-level DSC and ITC instruments that are specifically designed for life sciences and pharmaceutical sciences. These calorimeters are optimized for sensitivity and repeatability. They are power compensation calorimeters with cells that are fixed in place.
The company also manufactures isothermal and scanning calorimeters equipped with removable cells. The flexibility of removable cells allows samples that are solid, suspensions, slurries, or liquids to be investigated in the instrument. With a CSC isothermal calorimeter with removable cells, the same device can be used to study interactions of solutes with immobilized enzymes, tissue samples, or other solid materials in suspension, or to obtain analytical or kinetic data for slow reactions.
The Multi-Cell DSC (MC-DSC, CSC) is a workhorse calorimeter designed for a broad range of applications that are not possible to measure in the nanowatt-level DSC. The cell design of the device easily accommodates solid as well as liquid samples.The MC-DSC can collect data on three samples in a single experiment.
The company’s products range from power compensation calorimeters for looking at biopolymers in dilute solution to on-line temperature rise calorimeters used to solve production problems in the blowmolded plastics industry. The company also provides consultation, design, and the development of custom instruments for specific, specialized applications.
Mr. Sullivan is a freelance technical writer based in Hermosa Beach, CA, U.S.A. For more information, contact Calorimetry Sciences Corp., 890 W. 410 North, Ste. A, Lindon, UT 84042, U.S.A.; tel.: 801-763-1500; fax: 801- 763-1414. Now part of TA Instruments, e-mail: [email protected]; Web site: www.tainstruments.com.