Fluorescent chromophores fused to
specific proteins have greatly
increased our ability to image
dynamic events within the living
cell. However, conventional fluorescence images alone do not provide
much information about the
turnover rate and mobility of individual
molecules within a population.
Fluorescence recovery after
photobleaching (FRAP) is a technique
that has been used since 1976
to study diffusion by measuring the
rate of replacement of molecules
within a photobleached region of
the cell by fluorescent molecules
from neighboring locations.1 FRAP
measurements of diffusion rates and
mobility have yielded insights into
the organization of proteins in
receptor–ligand binding, macromolecular
complexes, and membrane
structure.
The green fluorescent protein
(GFP) has been mutated for optimal imaging and generally exhibits an
excitation peak coincident with the
commonly used 488-nm line of an argon laser and emissions that can
be collected by standard fluorescein
barrier filters. Further modification
of this protein has produced a
photoactivatable GFP (PA-GFP) in
which exposure to an intense light
pulse photoconverts the GFP
chromophore, causing a 100-fold
increase in the protein’s fluorescence.
2 Thus, only regions that
have been photoactivated will emit
above-background green fluorescence
when excited with 488-nm
laser light. Photoactivated GFP-tagged
proteins can then be tracked
as they move within the cell.
This recent derivation of PA-GFP
enables distinct pools of molecules
to be highlighted within live cells.
Using light microscopy, photoactivation
can be targeted to a defined
region within the field of view.
GFP-tagged proteins within the
targeted region can be monitored
by fluorescent imaging , while
GFP-tagged proteins located outside
the targeted region remain
only marginally fluorescent.
Thus, through the use of targeted
laser stimulation, both FRAP and
PA-GFP can be utilized as complementary
monitors of protein trafficking
in live cell imaging studies.
Laser scanning mechanisms
Most studies of the type described
here are performed using laser scanning confocal microscope systems.
Photobleaching is typically performed
using a high-intensity pulse
of the same laser employed to image
the fluorochrome: 488 nm in the
case of GFP, and 514 nm for yellow
fluorescent protein (YFP). Targeted
photoactivation of GFP is achieved
using brief exposure from a 405-nm diode laser or the 413-nm line of a krypton laser. In point scanning confocal microscopes, galvanometer-driven
mirrors scan the laser beam
over a rectangular field of view in a
raster pattern. For stimulation
(photoactivation or photobleaching),
the angle traversed by the mirrors
can be reduced in order to concentrate the photon flux of the
bleaching or activating laser. After a
period of stimulation, the mirrors are
returned to their full field of view for
conventional imaging. Due to the
time lag between the stimulus scanning
and image acquisition, rapid
cellular events may be missed before
imaging resumes.
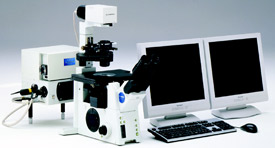
Figure 1 - FluoView FV1000 confocal laser scanning microscope.
The FluoView FV1000™ confocal
laser scanning microscope (Olympus
Corp., Tokyo, Japan) has
addressed this issue by adding a second,
independent scanner for laser
stimulation while the main imaging scanner continues to capture image
information before, during, and after
the stimulus (Figure 1). This simultaneous
(SIM) scanner is synchronized
in time and space with the
main scanner so that the SIM scanner
precisely stimulates the defined
regions of interest.
FRAP and PA-GFP used in
ion channel studies
Ion channels are organized in membrane
microdomains close to the signaling
molecules that interact with them.
Dynamic interactions such as protein
binding, turnover, and sequestration are
important mechanisms for modulating
ion channel activity. Understanding
these mechanisms is critical to the development
of therapeutic agents for neurological
disorders. The Tamkun Laboratory
at Colorado State University (Fort
Collins, CO) has used FRAP and PAGFP
to elucidate the different organization
and trafficking of functionally distinct
voltage gated potassium channels.3,4
Voltage gated ion channels are important
components of the electrical signaling
pathway in excitable cells such as neurons.
These channels open and close in
response to changes in transmembrane
voltage, allowing ions such as Na+, K+,
and Ca++ to enter or leave the cell.

Figure 2 - Rapid recovery of fluorescence in FRAP experiments using GFP-tagged Kv1.4. Similar FRAP
experiments using GFP-tagged Kv2.1 showed no visible recovery. Images from a FRAP series in a GFP-Kv1.4
expressing HEK cell. ROI#2 (red circle) was bleached using the 405-nm line of a FluoView FV1000 laser scanning
confocal microscope in Tornado Scanning mode and recovery monitored every 5 sec for 250 sec. ROI#3
(cyan line) was used for quantification (data not shown). All research images courtesy of Dr. Kristen M.S.
O’Connell and Prof. Michael M. Tamkun (Colorado State University).
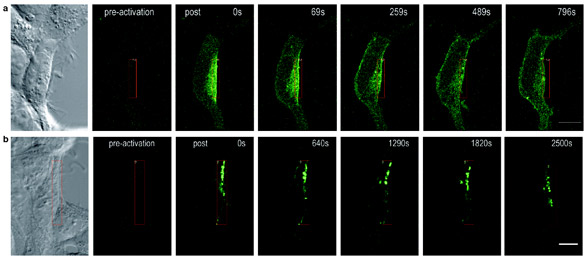
Figure 3 - Comparison of Kv1.4 and Kv2.1 mobility using PA-GFP. Scale bars: 10 μm. a) Rapid diffusion of activated PA-GFP-labeled channels from the
site of photoactivation throughout the membrane. Approx. 13 min after photoactivation, PA-GFP-Kv1.4 is evenly distributed over the entire cell membrane. b)
Slow organization of activated PA-GFP-labeled channels into discrete puncta and restricted movement to membrane sites outside of the activated region.
FRAP studies indicate that the channel
known as Kv1.4 is localized to the
plasma membrane and diffuses rapidly
(Figure 2). In contrast, Kv2.1 appears
to be less mobile within the membrane.
Using PA-GFP, Kv1.4 again
appears to diffuse rapidly to surrounding
areas of the membrane (Figure 3a),
whereas Kv2.1 organizes into puncta
that move slowly to adjacent membrane
(Figure 3b). Corroborative studies
indicate that Kv1.4 sediments as a
small tetramer, whereas Kv2.1 is part
of a large macromolecular complex.
Additional advantages
Along with the FluoView FV1000 SIM
scanner, a technical development known
as Tornado Scanning (Olympus) has
been added to address the bleaching limitations
of conventional raster scanning.
With ordinary raster scanners, the mirrors
must slow down and reverse direction
at the end of each line. This results
in an increased dwell time for the laser at
the edges of the scanned rectangle. To
counter this uneven exposure, the laser
beam is blocked outside of the field of
view, during the mirror turnaround.
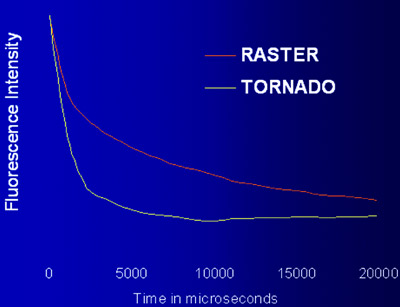
Figure 4 - Comparison of bleaching rates between Tornado Scanning and conventional raster
scanning using the same laser intensity on test targets.
Such beam-blanking at the edge of the
image is important during fluorescence
imaging. However, when beam-blanking
occurs during photobleaching studies, fluorescence
recovery can begin at multiple
time points, potentially confounding the
true kinetics of the cellular process. Tornado
Scanning, an option provided by the
Fluo View FV1000, is a continuous spiraling
scan, described by a circle drawn on
the image. The relentless laser exposure
results in a faster bleach rate (Figure 4).
The same loss of fluorescence occurs in a
shorter time, arguably reducing the overall
laser exposure for the cell.
Conclusion
Important to both FRAP and PA-GFP
experiments, rapid dynamic molecular
movements within living cells can now
be simultaneously observed during an
efficient stimulation process. Molecular
biology and optoelectronics technology are coming together to elucidate
the dynamic world of the living cell.
References
- Jacobson K, Derzko Z, Wu ES, Hou Y,
Poste G. Measurement of the lateral
mobility of cell surface components in
single, living cells by fluorescence recovery
after photobleaching. J Supramol
Struct 1976; 5(4):565(417)–76(428).
- Patterson GH, Lippincott-Schwartz J. A
photoactivatable GFP for selective photolabeling
of proteins and cells. Science
2002; 297:1873–7.
- O’Connell KMS, Tamkun MM. Role of
the N- and C-terminal domains of Kv2.1
in channel trafficking and cell surface
localization. 49th Annual Meeting of the
Biophysical Society, Long Beach, CA,
Feb 12–16, 2005.
- O’Connell KMS, Tamkun MM. Distinct
subcellular localization and trafficking
of Kv2.1, Kv1.4 and Kv1.3 channel
isoforms. 49th Annual Meeting of the
Biophysical Society, Long Beach, CA,
Feb 12–16, 2005.
Ms. Goodacre is Applications Specialist, Olympus
America Inc., 2 Corporate Park Dr.,
Melville, NY 11747, U.S.A.; tel.: 800-455-8236; fax: 631-844-5111; e-mail: [email protected]. Dr. O’Connell
is a Fellowship Grant Trainee in the laboratory
of Prof. Michael M. Tamkun, Department of
Biomedical Sciences, Colorado State University,
Fort Collins, CO, U.S.A.