In the current economic climate the pharmaceutical industry is focusing, more than ever, on the cost effectiveness and efficiency of drug discovery and development. Laboratory automation and high-throughput applications are being demanded for processes that are typically performed manually.
Enzyme kinetic measurements require finely timed and complex experiments to achieve the necessary accuracy. Kinetic measurements are needed to assess the Michealis-Menten (Km) constant, maximum rate of reaction (Vmax), and inhibition constant (Ki). Developments in the methodology and computing technology now mean that we have spectrophotometers that can perform the experiments with sufficient speed to provide accurate data for the calculation of these complicated constants.
Introduction to enzyme kinetics
The Michaelis-Menten or Km equation provides a constant that describes the rate of conversion of a substrate (the rate of reaction) for a specific enzyme-catalyzed reaction. The Km is defined as the concentration of substrate that elicits as the half-maximal (Vmax) rate of reaction. Essentially, this signifies the affinity of the enzyme for its substrate; a small value for Km suggests that the enzyme is biochemically matched to the particular substrate.
The Vmax of an enzyme-catalyzed reaction is generally considered to be the saturation point of the enzyme for the substrate, where any further addition of substrate cannot influence the rate of reaction due to substrate congestion of the active site. The Vmax is represented graphically when a plot of rate versus substrate concentration reaches a plateau. The Km and Vmax are both values that should remain constant for an enzyme, provided that the concentration of the enzyme does not change.
The inhibition constant (Ki) is a measure of how effective an inhibitor is at preventing catalysis of the reaction by the enzyme. The Ki of an inhibitor for its enzyme is determined experimentally by assessing the concentration of inhibitor required to reduce the Vmax of the reaction by half of the uninhibited reaction. A more effective inhibitor will reduce the Vmax at a lower concentration, possibly benefiting the patient by keeping doses low. It is in the evaluation of the Ki that the pharmaceutical industry needs the most sensitive and efficient methods for assessing enzyme kinetic activity.
Demands of modern industry
The pharmaceutical industry spends billions of dollars annually on the development of new drugs. Historically, some of the most effective pharmaceutical interventions have been through inhibition of an enzyme, e.g., the statin inhibition of the enzyme HMGCo-A reductase in the treatment of high cholesterol. As such, pharmaceutical measurement of enzyme activity in the laboratory plays a vital role in drug discovery and development.
The pharmaceutical industry today requires higher-throughput, more efficient screening methods to maintain the level of productivity in drug discovery and development. Adaptation to high-throughput format can result in a delay in measuring the initial rate of reaction, or not measuring the rate of reaction at sufficiently short intervals. These technical issues could lead to a loss in data quality and a reduction in the accuracy of the calculated enzyme constants. New technology has generated photometers that can read each well simultaneously; however, these machines are prohibitively expensive in terms of both the instrument itself and the computing power needed to maintain the data stream.
This article outlines the use of mutliple-well photometric and high throughput microplate equipment with a view to optimizing the efficiency of pharmaceutical enzyme kinetic activity measurements. To demonstrate the efficacy of these technologies, the authors adapted a photometric method for measuring β-galactosidase activity, both in the presence and absence of the inhibitors 2-phenylethyl β-dithiogalactoside (PETG) and D-lactose. β-galactosidase is an enzyme commonly used in the laboratory for gene expression activity measurements in cells, where the activity of the enzyme is used to indicate the activation of a transcription factor or signaling pathway.This study thus provides additional value as information for scientists wishing to perform these experiments in a higher-throughput format.
Materials and methods
Spectrophotometric instruments and measurement parameters
A Thermo Scientific Multiskan GO spectrophotometer (Thermo Fisher Scientific, Vantaa, Finland) with a 96- and 384-well format was used with the following experimental parameters: wavelength, 420 nm; half bandwidth, 2 nm; read time delay, 10 sec between reads of each well.
A Thermo Scientific Multiskan FC filter photometer with a 96- and 384-well format were used with the following experimental parameters: wavelength, 420 nm; half bandwidth, 6 nm; read time delay, 10 sec between reads of each well.
Microplates
The microplates used experimentally were selected for their assay compatibility and superior optical performance: 96-well, clear F-bottom plate; 384-well, F-bottom clear plate with square wells; and 384-well, clear F-bottom shallow-well plate.
Assay buffers and reagents
- Assay buffer: 80 mM Na-phosphate buffer, pH 7, supplemented with 1 mM MgCl2 and 50 mM β-mercaptoethanol
- Enzyme stocks: β-galactosidase from Escherichiacoli, aqueous solution with glycerol: specific activity 1300 U/mL; stock solution 100 IU/mL in water
- Substrate: ortho-nitrophenol by β-galactosidase (ONPG); stock solution 50 mM in water
- Inhibitors:
1) D-(+)-lactose monohydrate; stock solution 250 mM in water;
2) 2-PETG; stock solution 33.3 mM in methanol.
Assay condition optimization
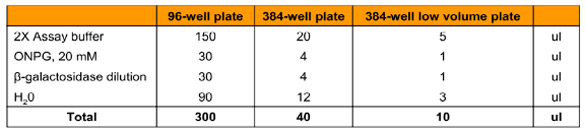
Table 1 - Enzyme titration assay compositions with different microplates
Assay conditions were optimized to determine the efficiency, sensitivity, and data quality of the assay once applied to a high-throughput format. To do this, a dilution series of β-galactosidase was prepared ranging from 0.02 to 10 IU/well, and the assay was performed in quadruplicate (Table 1). Once plated in the three microplate formats, the reactions were allowed to run for 60 min at 25 °C. Enzyme concentrations that produced a linear rate increase for the first 10 min of the reaction were selected and taken forward into future experiments.
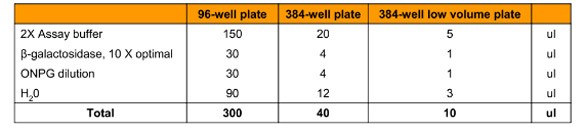
Table 2 - Substrate titration assay compositions for Km and Vmax determination with different microplates
Further optimization of assay conditions was performed by preparing a dilution series of ONPG running from 0.01 to 5 mM final concentrations (Table 2). Reactions were performed at 25 °C for 20 min; the ONPG concentrations that gave the greatest initial rate of reaction were selected for use in further reactions.
Determination of inhibitor activity
For experimental reasons, the activity of the inhibitors was established in 96-well format only. Dilution series of the two inhibitors were prepared as follows and are expressed as final concentrations:
- D-(+)-lactose monohydrate: 0.4 to 200 µM
- 2-PETG: 2 to 1000 µM
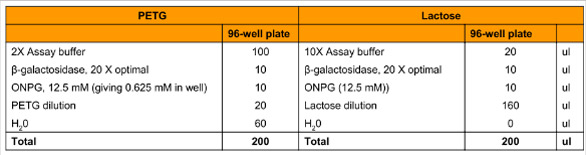
Table 3 - Inhibition assay compositions with different microplates
Table 3 outlines the optimization and setup of the assay to calculate the Ki for each inhibitor. Reactions were performed at 25 °C for 20 min, and the data were used to calculate Ki by Lineweaver-Burk plot.
Results and discussion
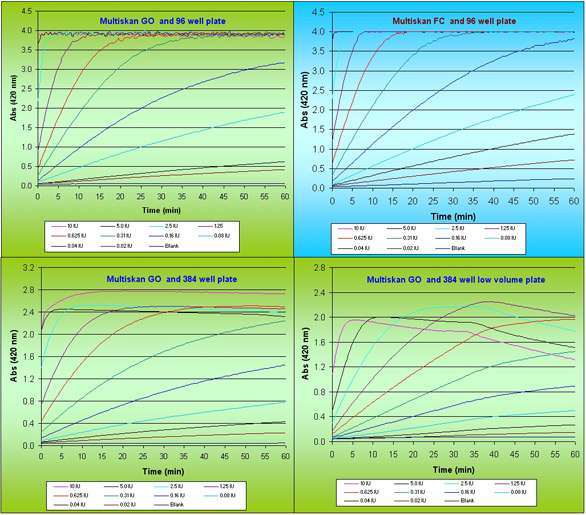
Figure 1- Kinetic curves of β-galactosidase enzyme reaction with different enzyme activities.
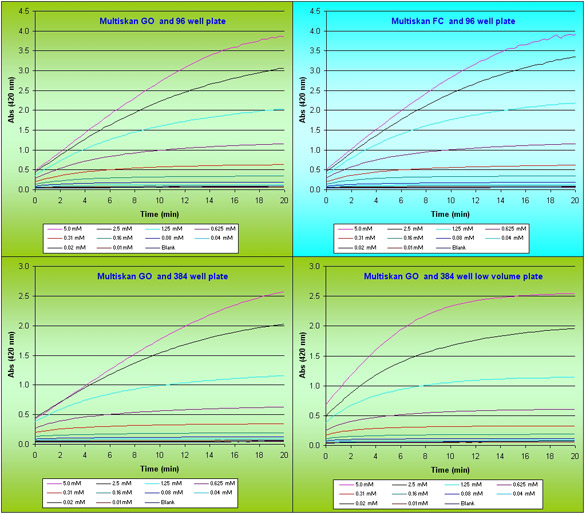
Figure 2 - Kinetic curves of β-galactosidase enzyme reaction with different substrate concentrations.
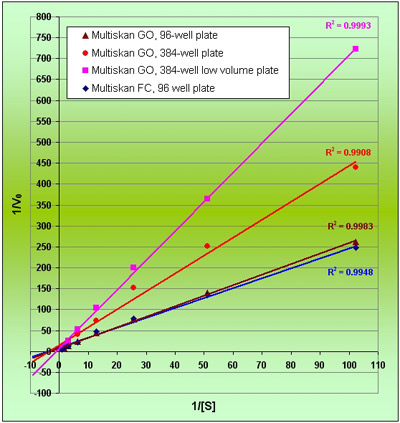
Figure 3 - Lineweaver-Burk plot of Km and Vmax.
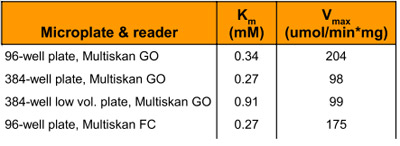
Table 4 - Calculated Michaelis-Menten parameters of the β-galactosidase reaction
Data quality for measurement of Km and Vmax
The determination and measurement of Km and Vmax requires large volumes of high-quality data, obtained in the early stages of the reaction.β-galactosidase activity plotted against reaction time for titration of enzyme concentration is shown in Figure 1. The adaptation of the assay for high-throughput format (96- and 384-well, respectively), does not appear to have affected the data quality in determining rate of reaction. As the reaction proceeds past 25 min in a 384 shallow-well format, the rate of reaction seemingly decreases; this is an artefact caused by the accumulation of product molecules in a low reaction volume. ONPG concentrations of 0.5 to 1 µM are optimal for a rapid rate of reaction and avoiding the problem of accumulated product.
Substrate concentration is a fundamental factor in considering the cost and efficiency of a high-throughput assay. Data showing the effect of substrate concentration are given in Figure 2. These experiments were performed at optimal concentrations of enzyme, as determined previously, and demonstrate that the use of microplates for assays of enzyme kinetics does not alter data quality if optimized adequately.
Km and Vmax are constants and should remain the same for a given concentration of an enzyme and substrate. These constants were calculated by the Lineweaver-Burk plot (1/Vo vs 1/[S]) (Figure 3); the values are shown in Table 4. The high throughput format of the assay has generated excellent data, and although literature comparisons are difficult (since reference values are measured at 30 °C), the data produced from the 96- and 384- well formats are broadly comparable with each other.
Determination of Ki
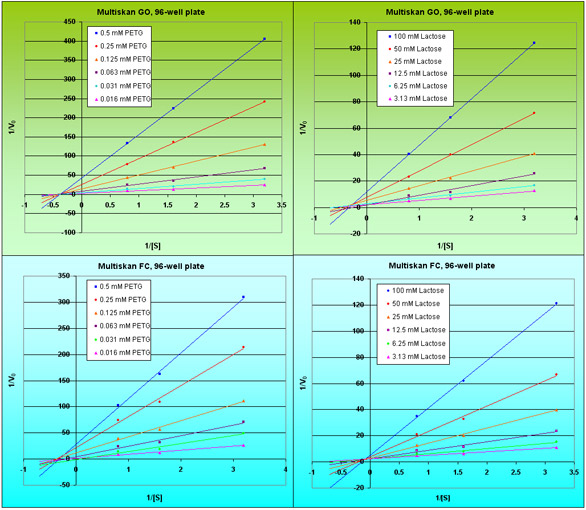
Figure 4 - PETG and lactose inhibition plots of the β-galactosidase enzyme reaction.
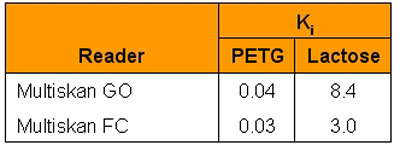
Table 5 - Inhibition constants of the β-galactosidase reaction
Ki is a measure of how effective an inhibitor is at preventing catalysis of the reaction by the enzyme. At given concentrations of enzyme and substrate, the value of Ki should remain constant and can be calculated by the Lineweaver-Burk plot (Figure 4). Values for Ki are shown in Table 5 in full. PEGT demonstrates excellent consistency across the two photometers used; however, D-lactose shows some small variation.
Conclusion
The results described in this paper show that high-quality, consistent data can be obtained from the high-throughput application of an enzyme kinetic analysis. The Thermo Scientific Multiskan GO and Multiskan FC have been demonstrated to be very robust. The technology provides the fast, repeated measurements needed for this type of analysis. This study highlights that enzyme kinetic analysis has moved forward to increase efficiency and cost effectiveness for the modern pharmaceutical industry.
The authors are with Thermo Fisher Scientific, Sample Preparation and Analysis, Ratastie 2, 01620 Vantaa, Finland; tel.: +358 9 329100; e-mail: [email protected].