Protein homogeneity is an important issue in many
fields of research, be it the characterization of newly
isolated molecules, the identification of crystallizable
samples, or the preparation of formulations for therapeutic
applications. In each case, the biological
material must be analyzed under conditions that do
not alter either its composition or its activity.
Protein heterogeneity
Many proteins share the characteristic of heterogeneity
with respect to mass, charge, or shape. The
causes of protein heterogeneity are multiple. Partial
post-translational modifications in vivo can be a
source when the protein molecules are overproduced
in another organism. Incomplete cleavage by proteolytic
enzymes during extraction from the cellular
medium is another one since it frequently generates
a mixture of polypeptide chains that differ by length
and mass. Because of the presence of charged amino
acid residues, the heterogeneity in mass is frequently
accompanied by a heterogeneity in charge. As a consequence,
oligomeric proteins formed by several subunits
can exhibit complex patterns upon separation
according to hydrodynamic or electric properties.
Finally, many unmodified proteins have a propensity
to aggregate when they are very pure. Aggregation
involves either hydrophobic or ionic interactions or
disulfide bridge formation, and the aggregates are
generally highly polydisperse in size. In some
instances they can be in equilibrium with the
monomeric form of the protein. The study of the
structural features of such protein particle populations
requires noninvasive analytical methods.
Protein aggregation detection
methods
Great charge differences are often detectable by ion exchange chromatography, but more subtle ones may be visualized only by gel electrophoresis or isoelectric focusing. Most often, these approaches are insufficient to confirm either the presence or absence of aggregates and they alter the sample.
Major differences in particle mass, volume, or shape
can usually be monitored by fractionating the sample's
content on a size exclusion chromatography column
or in an ultracentrifuge. These methods have the disadvantage
that nonspecific and loose aggregates can
be disrupted by the shear forces resulting from solvent
flow around the beads composing the column or by
the pressure created in the gravity field. On the other
hand, field flow fractionation provides a gentler separation
of the macromolecular components. Further,
spectroscopic methods are a good alternative to gain
insight into the composition of a mixture.
Dynamic light scattering (DLS), also called quasielastic
light scattering (QELS) or photon correlation
spectroscopy (PCS), is the least invasive of all the
analytical methods. Scientists at the Institute for
Molecular and Cellular Biology (IBMC) (Strasbourg,
France) have been working with the DynaPro instrument (Wyatt Technology, Santa Barbara, CA) for a
decade. Sedimentation of the dust particles and large
aggregates that negatively affect the light scattering
measurements is the method of choice, as opposed to
eliminating them by filtration because filter membranes
may not be entirely inert and may adsorb
charged or hydrophobic particles.
In practice, the sample (12 μL of solution at 1–2
mg/mL for a protein with a relative mass of 50,000)
is transferred to a square quartz cuvette that is centrifuged
for 10 min at 4500 rpm in a tabletop centrifuge.
The cuvette is then placed in the temperature-controlled holder of the DynaPro and it is
irradiated by the visible beam of a laser. The small
fraction of the incident photons that is scattered by
the particles in solution is collected at a fixed angle
and the signal is amplified by an avalanche diode.
The variation of the signal over time is analyzed by a
correlator and is used to generate an autocorrelation
curve. The autocorrelation function is calculated
from the latter by exponential regression and the
translational diffusion coefficient (D) of the particles
from which the variance is derived. Assuming that
the particles are hard spheres with a known density,
their hydrodynamic radius can be calculated and the
apparent molecular mass extrapolated.
The variance on D gives the polydispersity on the radius.
On average, a measurement takes approximately 12 sec,
and valuable statistics are typically obtained with a set of
two dozen measurements. The value of the baseline and
the sum of the squares characterizing the regression are
important indicators of the quality of the data. Moreover,
the polydispersity is an invaluable piece of information in
addition to the particle parameters. Since proteins are
never dissolved in pure water, corrections must be
applied to take into account the composition of the solvent.
In particular, the solvent viscosity and refractive
index must be known. At any moment the data can be
recalculated with the help of a list of standard solvents
that is provided in a separate file, and the histogram of
the size distribution of the particles can be displayed.
A primary advantage of the DynaPro is that the samples
are contained in cuvettes, and thus they can be
recovered easily. Secondly, chemicals (i.e., detergents,
salts, or reducing agents) can be added to the sample
in order to test their effects on the protein aggregates.
Also, biochemicals such as ligands (e.g., substrates or
inhibitors) can be assayed to monitor their influence
on the degree of aggregation of enzymes or receptors.
Results
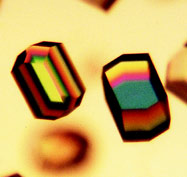
Figure 1 - Crystallographic and biochemical analyses have shown
that the best crystals of hen egg-white lysozyme can be grown in vitro
only if the protein is free of macromolecular impurities and aggregates.
At the IBMC, focus is placed on the study of the crystallogenesis
of proteins and their complexes with nucleic
acids. The goal is to find solvent conditions under which
these entities crystallize, and crystals that are of the best
quality for structural studies by X-ray or neutron crystallography
(Figure 1). A DLS analysis is routinely performed
prior to setting up crystallization assays in addition
to specific activity assays, as well as electrophoresis, size exclusion chromatography, and ultracentrifugation
analyses. The reason for this is that monodisperse protein
samples crystallize more often and produce better crystals
than polydisperse ones. DLS can thus provide important
information on the possibility of obtaining crystals. It can
also be used to search for an adequate solvent instead of
setting up a large number of useless crystallization assays.
Two striking examples illustrate the power of DLS measurements.
First, it is possible to show within a few minutes
that a single cycle of sample freezing is sufficient to
produce protein aggregates. After freezing at –20 °C, a
sample that was initially monodisperse became polydisperse.
Second, it was surprising to discover that some
proteins can be subjected to a tremendous aggregation
(that is invisible to the naked eye) after the addition of
a low concentration of a nonionic or a zwitterionic
detergent (such as beta-octylglucoside, lauryldiamino
oxide, and 3-[(3-cholamidopropyl)dimethylammonio]-
1-propanesulfonate [CHAPS]). This was all very unexpected
because these compounds are exclusively known
as solubilizing agents of protein aggregates. All these
DLS measurements were performed in less than an hour
and were reproducible.
Conclusion
DLS is an indispensable tool for the accurate characterization
of the state of proteins or nucleoprotein
complexes in solution. It is a simple, rapid, and noninvasive
method for verifying the presence of aggregates
in biological samples.
Dr. Lorber is Senior Scientist, Crystallogenesis Group, Dept.
UPR9002, Institute for Molecular and Cellular Biology of
CNRS, F-67084 Strasbourg, France; tel.: +33 3 8841 7008;
fax: +33 3 8860 2218; e-mail: [email protected].