Inadequate absorption and bioavailability often make it difficult to deliver the drug to the target in vivo and pose significant challenges to the discovery and development of new drugs. A number of delivery and formulation technologies can be used to improve the solubilization and absorption of drug candidates. However, many of these approaches require a significant amount of material and are not suitable for application in the early drug-discovery phase, where the availability of compound can be limited. Formulation strategies that can be applied on the small scale can help to identify and address critical issues early in the drug-discovery process.
Drug nanosuspensions are a promising approach to formulation1–5 and consist of crystalline drug particles that are generally <400 nm in diameter suspended in aqueous solution. The nanocrystalline particles have a large surface area relative to their overall sizes, which results in a dramatically increased rate of dissolution, improved absorption rates and increased bioavailability.1,4,6 Due to their small particle size, nanosuspensions are amenable to a variety of administration routes, including oral delivery and direct systemic delivery via parenteral action or inhalation.7,8
Nanoparticle formulations have been used successfully in the development and commercialization of several drug products.9–11 However, it has been difficult to translate this success into the drug discovery space, where it would be used to support the evaluation of multiple drug candidates, due to the often prohibitive amount of material and time required to identify suitable nanosuspension formulations. The high-throughput drug-sparing technology described in this article enables the application of optimized nanosuspension formulations in early drug discovery.12
Materials
Reagents and solvents were obtained from commercial sources and used as received unless otherwise noted. Naproxen was obtained from commercial sources and used as received.
Screening of nanosuspensions using acoustic mixing
A UV-Star clear, flat-bottom 96-well plate (Greiner Bio-One GmbH, Monroe, N.C.) with a total well volume of 350 μL was used as the mixing container. The wells were charged with 500 μm YTZ grinding media (800 mg) from Tosoh (Grove City, Oh.). Drug solid (2 mg) was added to each well. Water (136 μL) was mixed with 10 μL of a 5% w/w stock solution of the appropriate polymer and 2 μL of a 1% stock solution of the appropriate surfactant as necessary. The aqueous mixture was then added to each well to form a drug suspension. Next, the 96-well plate was sealed using a PlateLoc thermal sealer (Velocity11, Palo Alto, Calif.) and mixed with the LabRAM Resonant Acoustic mixer (Resodyn Acoustic Mixers, Inc., Butte, Mont.) at 40% intensity (~50 g acceleration) for up to 90 minutes. A 5-μL aliquot of the nanosuspension was taken and diluted to approximately 1 mg/mL concentration with water for particle size analysis.
Nanoparticle size analysis
Particle size measurements of the nanosuspensions were obtained using a DynaPro Plate Reader (Wyatt Technology Corp., Santa Barbara, Calif.), which performs dynamic light scattering (DLS) in situ in standard 96-, 384- or 1536-plates. DLS is a rapid technique that analyzes the fluctuations of light scattering intensity due to Brownian motion to determine the diffusion coefficients of suspended nanoparticles. The diffusion coefficients are converted to a measure of size known as hydrodynamic radius, Rh, via the Stokes-Einstein relation, Rh = (kB T)⁄(6πηDt), where kB is the Boltzmann constant, T is the absolute temperature, π is the viscosity of the solvent and Dt is the measured diffusion coefficient. The DynaPro Plate Reader’s size monitoring ranges from 0.5 to 1000 nm (radius). An example of a DLS nanoparticle size distribution is shown in Figure 1.
Figure 1 – Typical particle size distribution obtained using the regularization algorithm to analyze dynamic light scattering measurements.DLS data may be analyzed in two ways: the cumulants method provides an average size plus the polydispersity (distribution width), while the regularization method calculates a complete size distribution, albeit with relatively low resolution. DYNAMICS software, provided with the DynaPro Plate Reader, automates the measurements and performs all the necessary calculations. After analysis, the particle size of the samples can be color-coded to display a heat map of the formulation compositions that give the smallest particle size (Figure 2).
Figure 2 – Heat map showing the particle size of various nanosuspension formulations prepared in a 96-well plate at four time points analyzed in a 384-well plate using the DYNAMICS software on data acquired from the DynaPro Plate Reader. Green indicates smaller particle size, red larger particle size and black the absence of nanoparticles (particles >1 μm).The nanosuspension samples were diluted to approximately 1 mg/mL concentration, and a 25-μL aliquot was dispensed into a low-volume, black polystyrene 384-well plate for analysis (Corning, Corning, N.Y.). The particle size of each sample was reported as an average of 10 acquisitions with an acquisition time of 3 seconds at 25 °C. The autocorrelation curves obtained were fitted and the average particle diameter, D50, and D90 were determined using the cumulants or regularization model when appropriate.
Results and discussion
Drug nanosuspension formulations are often prepared using a top-down approach where the size of the drug particles is reduced to the nanometer scale in an aqueous suspension through the application of mechanical energy in media milling.1,13–15 In these formulations, additional additives such as polymers and surfactants are typically required to stabilize the nanoparticles by adsorbing to its surface and providing a steric or ionic barrier to inhibit aggregation or agglomeration.1,13–17 However, it is often difficult to determine the identity and concentration of the additive required, and the use of a nonoptimal stabilizer may result in inefficient milling or poor stability of the nanoparticles. As a result, formulation optimization is typically empirical and requires the evaluation of multiple formulation conditions, which can be a materials- and time-consuming process.16
Figure 3 – Schematic showing the resonant acoustic mixing process, which results in more uniform transfer of energy homogeneously throughout the sample. (Image courtesy of Resodyn Acoustic Mixers.)To address this challenge, a procedure that screens and optimizes nanosuspensions in a high-throughput manner was developed using the Resonant Acoustic mixing system.18–21 In this technique, low-frequency, high-intensity acoustic energy is added to the container to homogeneously mix the contents in a low-shear gradient (Figure 3). Since the mixing process is applied homogeneously, consistent results can be observed for a wide range of different containers.
Using this technique, a high-throughput approach was developed using a standard 96-well plate. The procedure requires only 1–2 mg of compound per well. For application in early discovery, where the available amount of drug is often limited, a small amount of drug can be used to evaluate a number of formulation options (e.g., assessing five different formulation combinations would require <10 mg of drug). Later, a full optimization screen using the entire 96-well plate map design can be leveraged when compound availability is no longer a critical issue, facilitating a more comprehensive exploration of the optimal space for a nanosuspension formulation.
While the generation of a large number of drug nanosuspension formulations can now be undertaken rapidly, the analysis of the resulting products becomes a critical rate-limiting step. The characterization of the final nanoparticle size is used to identify the optimal formulation composition. To accommodate the high-throughput screening procedure, a complementary high-throughput analytical technique that uses a minimal amount of sample is required.
Table 1 – Average particle size and polydispersity of naproxen nanosuspensions prepared using acoustic mixing in a 96-well plate
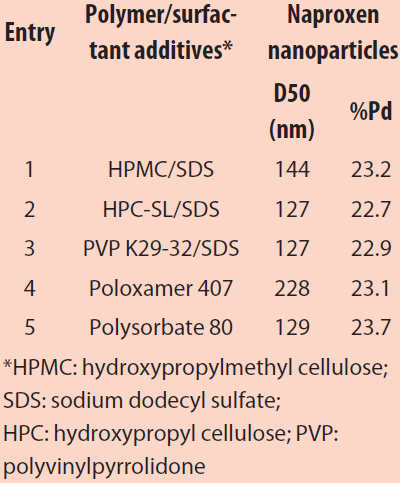
High-throughput characterization of nanoparticle size can be accomplished by DLS with the DynaPro Plate Reader. In this method, a small aliquot of the nanosuspension formulations (e.g., 5 μL) was removed from the 96-well plate and diluted in water to an appropriate concentration, typically 1 mg/mL, for analysis by DLS. The sample was then transferred to a 384-well plate for analysis on the DynaPro Plate Reader. The small amount of sample required for DLS analysis enables the evaluation of particle size at multiple time points to track the milling efficiency of a formulation over time (typically four time points). The DLS analysis time is very rapid, only 30 seconds per sample. The optimal formulation composition can then be quickly identified by means of the DLS heat map, which is set to display the desired particle size range in green (Figure 2).
Naproxen was nanomilled using this screening approach. As seen in Table 1, a range of nanoparticle sizes was obtained depending on the polymer stabilizer system used. While smaller nanoparticles were observed with entries 2–3, significantly larger particle sizes and particle distributions were seen with entry 4 using poloxamer 407 as a neutral surfactant. The optimal formulation compositions can thus be quickly identified based on reduced particle size as well as monomodal particle size populations.
Conclusion
Suspensions of drug nanoparticles are a particularly attractive formulation option in the drug discovery space. Optimization of a drug nanosuspension formulation requires careful consideration of the polymer or surfactant additive used to stabilize the nanoparticles. Resonant acoustic mixing can generate a large number of different nanosuspension formulations in parallel with a minimal amount of material using a 96-well plate. The high-throughput analysis of the resulting particle sizes can then be used to rapidly identify the optimal composition for the formulation. This approach can facilitate the use of nanosuspension formulations to support early studies in drug discovery and to address challenges with new drug candidates.
More information on the companies mentioned in this article is available on their websites. More information on the DynaPro Plate Reader is available from Wyatt Technology or at www.wyatt.com/DynaPro.
References
- Merisko-Liversidge, E. and Liversidge, G.G. Nanosizing for oral and parenteral drug delivery: a perspective on formulating poorly water-soluble compounds using wet media milling technology. Adv. Drug Deliv. Rev. 2011, 63, 427–40.
- Pu, X.; Sun, J. et al. Formulation of nanosuspensions as a new approach for the delivery of poorly soluble drugs. Curr. Nanosci. 2009, 5, 417–27.
- Merisko-Liversidge, E.M. and Liversidge, G.G. Drug nanoparticles: formulating poorly water-soluble compounds. Toxicologic Pathology 2008, 36, 43–8.
- Kesisoglou, F.; Panmai, S. et al. Nanosizing—oral formulation development and biopharmaceutical evaluation. Adv. Drug Deliv. Rev. 2007, 59, 631–44.
- Rabinow, B.E. Nanosuspensions in drug delivery. Nat. Rev. Drug Discov. 2004, 3, 785–96.
- Noyes, A.A. and Whitney, W.R. The rate of solution of solid substances in their own solutions. J. Am. Chem. Soc. 1897, 19, 930–4.
- Sun, B. and Yeo, Y. Nanocrystals for the parenteral delivery of poorly watersoluble drugs. Curr. Opin. Solid State Mater. Sci. 2012, 16, 295–301.
- Muller, R.H.; Gohla, S. et al. State of the art of nanocrystals—special features, production, nanotoxicology aspects and intracellular delivery. Eur. J. Pharm. Sci. 2011, 78, 1–9.
- Shen, L.J. and Wu, F.-L. Nanomedicines in renal transplant rejection—focus on sirolimus. Int. J. Nanomed. 2007, 2, 25–32.
- Wu, Y.; Loper, A. et al. The role of biopharmaceutics in the development of a clinical nanoparticle formulation of MK-0869: a beagle dog model predicts improved bioavailability and diminished food effect on absorption in human. Int. J. Pharm. 2004, 285, 135–46.
- Oliver, I.; Shelukar, S. et al. Nanomedicines in the treatment of emesis during chemotherapy: focus on aprepitant. Int. J. Nanomed. 2007, 2, 12–18.
- Leung, D.H.; Lamberto, D.J. et al. A new and improved method for the preparation of drug nanosuspension formulations using acoustic mixing technology. Int. J. Pharm. 2014, 473, 10–19.
- Eerdenbrugh, B.V.; Van den Mooter, G. et al. Top-down production of drug nanocrystals: nanosuspension stabilization, miniaturization and transformation into solid products. Int. J. Pharm. 2008, 364, 64–75.
- Muller, R.H.; Moschwitzer, J. et al. Manufacturing of Nanoparticles by Milling and Homogenization Techniques. Taylor & Francis Group LLC: New York, NY, 2006; Vol. 159, pp 21–51.
- Merisko-Liversidge, E.; Liversidge, G.G. et al. Nanosizing: a formulation approach for poorly water-soluble compounds. Eur. J. Pharm. Sci. 2003, 18, 113–120.
- Van Eerdenbrugh, B.; Vermant, J. et al. A screening study of surface stabilization during the production of drug nanocrystals. J. Pharm. Sci. 2009, 98, 2091–2103.
- Peltonen, L. and Hirvonen, J. Pharmaceutical nanocrystals by nanomilling: critical process parameters, particle fracturing and stabilization methods. J. Pharm. Pharmacol. 2010, 62, 1569–79.
- Lim, J.-Y. and Moon, J.-D. Multiphase Mixing Apparatus Using Acoustic Resonance. U.S. Patent 6,283,626 B1; Sept. 4, 2001.
- Coguill, S.L.; Cook, A.M. et al. Apparatus and Method for Resonant-Vibratory Mixing. U.S. Patent 7,188,993 B1; Mar. 13, 2007.
- Howe, H.W.; Warriner, J.J. et al. Method for Resonant-Vibratory Mixing. U.S. Patent 7,866,878 B2; Mar. 13, 2007.
- Mcpherson, M.D. Propellant and Explosives Production Method by Use of Resonant Acoustic Mix Process. Eur. Patent 2215033 A1; Nov. 25, 2010.
Dennis Leung is principal scientist, Timothy Rhodes is senior principal scientist and Annette Bak is director, Merck & Co., Inc., 126 E. Lincoln Ave., P.O. Box 2000, Rahway, N.J. 07065, U.S.A.; tel.: 732-594-6982; fax: 732-594-4858; e-mail: [email protected]; www.merck.com