Heavy metal pollution in water has been a public concern for decades. Heavy metals, which include lead, cadmium, mercury, chromium, zinc, copper, and nickel, are highly toxic with hidden pollution, cannot degrade in the environment, and can accumulate in the biological chain. These metals are distributed widely in the environment and are responsible for several diseases. Heavy metals have been ranked as an environmental priority pollutant in many countries. Therefore, it is important to monitor the levels of heavy metals in water, food, and other samples.
Several analytical techniques, such as inductively coupled plasma-optical emission spectrometry (ICP-OES), inductively coupled plasma-mass spectrometry (ICP-MS), spectrophotometry, and atomic absorption spectrometry (AAS), are available for the determination of heavy metals with sufficient sensitivity for most applications.1 However, it is difficult to directly determine extremely low concentrations of the required trace elements by these methods without preconcentration and separation. The limitations are associated not only with the insufficient sensitivity of these techniques, but also with matrix interference. For this reason, preliminary preconcentration and separation of trace elements from matrix are often required.
The most widely used techniques for the separation and preconcentration of trace elements include liquid–liquid extraction,2–4 coprecipitation,5–8 ion exchange,9 cloud point extraction,10–13 and solid-phase extraction (SPE).14–18 Among these, SPE has become increasingly popular compared to classical preconcentration and separation methods. SPE offers a high enrichment factor, rapid phase separation, is low in cost, consumes less organic solvent, and is able to be combined with different detection techniques in on- or offline mode.
As a new solid-phase extractant, nano-calcium titanate has been applied successfully in separation and enrichment.19,20 However, because of the small granules of nano-calcium titanate powder, it is difficult for it to subside in water, is easily lost, and is difficult to recycle. Thus, loading the nano powder in the carrier or making porous material is an effective way to solve this problem.21–25 However, the loaded powder particles can easily fall off and flow away during use, resulting in a decline in adsorption capacity.
In contrast, transforming the adsorbents into porous materials is more advantageous. In the authors’ previous studies, porous nano-barium strontium titanate by reversed-phase suspension polymerization and sol–gel method was prepared25 and achieved a satisfactory result. However, nano-barium strontium titanate is costly, thus restricting its application in practice. Compared to barium strontium titanate, calcium titanate is lower in cost for preparing sources and possesses high adsorption ability for heavy metal ions. However, the reversed-phase suspension polymerization and sol–gel method used for the preparation of porous nano-calcium titanate (PCTO-RSP) has not been studied.
In this work, PCTO-RSP was prepared successfully by reversed-phase suspension polymerization and the sol–gel method and a new porous block adsorbent was obtained. The adsorptive potential of adsorbent for the preconcentration of trace lead, cadmium, zinc, copper, and nickel ions was assessed. A new method using porous nano-calcium titanate as solid-phase extraction agent was developed for the preconcentration of trace lead, cadmium, zinc, copper, and nickel in water sample analyses by flame atomic absorption spectrometry (FAAS).
Experimental
Apparatus
A WYX-9003A atomic absorption spectrometer (Shenyang Yi Tong Analytical Instrument Co., Ltd., Shen yang, China) equipped with hollow cathode lamps for lead, cadmium, zinc, copper, and nickel as the radiation source was used for analysis. The operating conditions are given in Table 1.
Table 1 – FAAS operating conditions
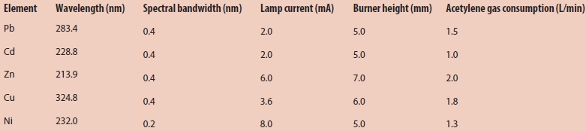
The pH values were measured with a PHS- 3C acidometer (Shanghai REX Instrument Factory, Shanghai, China) supplied with a combined electrode. A constant temperature bath shaker (Jin tan Experiment Instrument Factory, Jiangsu, China) was used for adsorption and desorption experiments.
The X-ray diffraction (XRD) diagram of synthetic material was measured with a D/max-RB X-ray diffractometer (Rigaku Corp., Tokyo, Japan). The morphology of the material was taken using an S-3400N scanning electron microscope (Hitachi, Tokyo, Japan).
Materials and reagents
The reagents used—calcium acetate, isopropyl alcohol, phenyl hydroxide, normal heptane, formaldehyde, glacial acetic acid, nitric acid, and ammonium hydroxide—were of analytical grade. Tetrabutyl titanate was chemically pure. Stock solutions of lead, cadmium, zinc, copper, and nickel were prepared by dissolving each metal (purity > 99.99%) in a small amount of 1+1 nitric acid separately and diluting to 1L with 0.1% nitric acid. Working standard solutions were prepared by appropriate dilution of the stock standard solutions. The water used in this study was distilled water.
Preparation of PCTO-RSP
First, a specific amount of tetrabutyl titanate was dissolved in isopropyl alcohol. After mixing for 0.5 hr, under the condition of continuous stirring, glacial acetic acid was added drop by drop, and the mixture was stirred until transparent. Subsequently, a specific amount of calcium acetate was directly added to this solution on a magnetic stirrer and the solution returned to clear. Ammonium hydroxide solution was then added to the precursor solution to adjust the pH value of 3.5. Thus, calcium titanate sol was synthesized. Mixing the sol and phenyl hydroxide, the mixture was added to normal heptane, mixed well, formaldehyde was added, and the solution was heated to 60 °C. The mixture was allowed to react for 1.5 hr and was placed in a drying oven at 90 °C. Subsequently, the dried gel was burned at 900 °C for 2 hr under an atmosphere of air and allowed to cool to room temperature. PCTORSP was immersed in 1 mol/L HNO3 for 15 min, neutralized by washing with water, dried at 105 °C, and stored in a desiccator.
General procedure
A specific amount of metal ion solution was placed into a 50-mL calibrated beaker flask with plug. The pH was adjusted to 6.0 with nitric acid or ammonia solution. After dilution to the mark with water, 0.1 g of PCTO-RSP was added. Covered with a plug, the beaker flask was shaken for 20 min in a constant-temperature bath shaker (200 rpm). The supernatant fluid was then inhaled directly to the atomic absorption spectrometer, where the concentrations of lead, cadmium, zinc, copper, and nickel ions were determined by FAAS. The adsorption capacity can be calculated as follows:
Where qe is the equilibrium adsorption capacity (mg/g), V1 is the solution volume (L), C0 is the initial concentration (mg/L), Ce is the equilibrium concentration (mg/L), and W is the mass of adsorbent (g).
The PCTO-RSP was then washed with water and the adsorbed metal ions were eluted with 5 mL of 0.5 mol/L HNO3 solution by shaking for 5 min. The concentrations of the metal ions in the eluents were determined by FAAS. The recycling rate was calculated as follows:
Where n is the elution recycling rate (%), V2 is the eluent volume (L), and C is the metal ion concentration (mg/L) in the eluent.
Results and discussion
Characterization of PCTO-RSP
The XRD pattern for the PCTO-RSP is shown in Figure 1. As can be seen, the sharp peaks at 23.26, 33.14, 47.56, 59.28, and 69.46 can be attributed to calcium titanate. It can be concluded that the synthetic material made of calcium titanate had a perovskite structure. According to the Scherrer equation, the average grain diameter of calcium titanate crystal is 32 nm.
Figure 1 – XRD pattern of PCTO-RSP.The scanning electron microscope (SEM) image of the PCTO-RSP is shown in Figure 2. The image shows that calcium titanate is porous block.
Figure 2 – SEM image of PCTO-RSP.Effect of pH on adsorption
Since the pH value of adsorption medium influences the active sites on the adsorbent surface, changes in pH value of adsorption medium will change the electric charges on the adsorbent surface, thus affecting the performance of adsorbing function groups of the adsorbent.20 In order to evaluate the effect of pH, the pH values of the solution were adjusted to pH 1–9 with nitric acid and ammonia solution, respectively. The results of the effect of pH on the adsorption percentage of studied ions are shown in Figure 3. It can be seen that the adsorption rates of Pb2+, Cd2+, Zn2+, Cu2+, and Ni2+ on the PCTO-RSP were high and pH dependent. The adsorption percentage of Pb and Zn at pH 4–9, Cd and Cu at pH 5–9, and Ni at pH 6–9 was 100%. In order to adsorb these metals simultaneously and avoid the hydrolysis of metal ions under high pH value, the pH value used in the experiment was 6.
Figure 3 – Effect of pH on the adsorption of the heavy metal ions on PCTO-RSP.Effect of shaking time
The adsorption capacities for Pb2+, Cd2+, Zn2+, Cu2+, and Ni2+ were determined using different shaking times (Figure 4). The results indicate that the adsorption capacity increased with an increase in shaking time and reached equilibrium at 10 min for Pb and Zn, 15 min for Cu and Ni, and 20 min for Cd. Therefore, in order to adsorb Pb2+, Cd2+, Zn2+, Cu2+, and Ni2+ together, the shaking time used in this study was 20 min.
Figure 4 – Effect of shaking time on the adsorption of the heavy metal ions on PCTO-RSPStatic adsorption capacity
According to the data in Figure 4, with 20 min of shaking and adsorption, the static adsorption capacities of PCTO-RSP to Pb2+, Cd2+, Zn2+, Cu2+, and Ni2+ reached 230.22, 32.45, 66.03, 88.09, and 23.91 mg/g, respectively. The adsorption amounts are all higher than those of nano-calcium titanate powder and the porous nano-barium strontium titanate by reversed-phase suspension polymerization and sol–gel method.20,25
Elution of the adsorbed metal ions
As shown in Figure 3, the adsorption of heavy metal ions at pH ≤1 can be negligible. Thus, after the heavy metal ions were adsorbed, 5 mL of HNO3 (0.05 mol/L–2.5 mol/L) were used for elution. The results show that recovery increased with an increase in HNO3 concentration. When the concentration of HNO3 was higher than 0.2 mol/L, the recoveries were up to 97%. In order to fully elute, 5 mL–0.5 mol/L nitrate solution was selected as the eluent.
After elution, the PCTO-RSP was washed with water, dried, and reused 10 times. Its adsorption performance did not decrease, indicating that this adsorption agent was very stable.
Enrichment factor and detection limit
To explore the possibility of enriching low concentrations of analytes from large volumes, the effect of sample volume on the retention of heavy metal ions was also studied. For this purpose, 25, 50, 100, 250, 500, and 1000 mL of sample solution containing 20 μg of Pb2+ and 5 μg of Cd2+, Zn2+,Cu2+, and Ni2+, respectively, were used. After adsorption, the samples were eluted with 5 mL–0.5 mol/L nitrate solution. The results show that the recoveries were reduced with the increase in solution volume. When the solution volume was 1000 mL, the recoveries were all above 92%. The enrichment factor was 200. The detection limits were calculated using the concentration of three times standard deviation, calculated from 11 runs of the blank solution with concentrations of Pb2+, Cd2+, Zn2+, Cu2+, and Ni2+ at 0.09, 0.011, 0.007, 0.006, and 0.031 μg/L, respectively.
Effects of coexisting ions
To investigate the influence of components on adsorption medium coexisting with heavy metal ion, potential interfering ions were added to solutions of 0.2 mg/L Pb2+ and 0.02 mg/L Cd2+, Zn2+, Cu2+, and Ni2+. The experimental results show that recoveries of the target analytes remained above 95%, even in the presence of ions in the following concentrations: 1000 mg/L for Na+, K+, SO42-, NH4+, and NO3-; 500 mg/L for Al3+, Ca2+, and Mg2+; 100 mg/L for PO43- and Cr(VI); 50 mg/L for Cr(III), Co2+; and 20 mg/L for Fe3+ and Ag+. These showed that amounts of ions did not have significant interference of the adsorption and recycling, indicating that the method has a high tolerance to matrix interferences.
Application
Surface water samples from the Liao He River, Hun He River, and Nan Hu Lake (Shenyang, China) were filtered through a 0.45-μm membrane filter. Also included was a tap water sample collected from the water supply of Shenyang City. According to the experimental method, Pb2+, Cd2+, Zn2+, Cu2+, and Ni2+ were determined, respectively. Recovery tests were conducted as well. The results (Table 2) indicate that the recoveries were reasonable for trace analysis, in the range of 92.28%–102.32%.
Table 2 – Determination of heavy metal ions in water samples
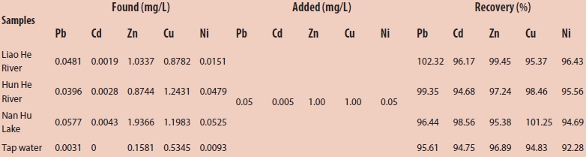
Conclusion
Porous nano-calcium titanate by reversed-phase suspension polymerization was prepared successfully and a new porous block adsorbent was used for heavy metal ion preconcentration. Lead, cadmium, zinc, copper, and nickel were quantitatively retained at pH 6–9. The adsorption capacities of PCTO-RSP for Pb2+, Cd2+, Zn2+, Cu2+, and Ni2+ were 230.22, 32.45, 66.03, 88.09, and 23.91 mg/g, respectively. The adsorbent can be regenerated completely by elution with 0.5 mol/L HNO3.
The adsorbent has been used for preconcentration from various aqueous solutions. The proposed method based on preconcentration with PCTO-RSP and determination by FAAS demonstrated enough sensitivity for those trace heavy metal ions in water determinations with satisfactory results.
References
- State Environmental Protection Administration of China. Analysis Method of Water and Waste Water, 4th ed., China Environmental Science Press, Inc.: Beijing, 2002; pp 286–415.
- Pena-Pereira, F.; Lavilla, I. et al. Spectrochim. Acta Part B 2009, 64, 1–15.
- Sereshti, H.; Khojeh, V. et al. Talanta 2011, 83, 885–90.
- Farajzadeh, M.A.; Bahram, M. et al. J. Hazard. Mater. 2009, 161, 1535–43.
- Bulut, V.N.; Ozdes, D. et al. Anal. Chim. Acta 2009, 632, 35–41.
- Tuzen, M.; Soylak, M. J. Hazard. Mater. 2009, 162, 724–9.
- Soylak, M.; Aydin, A. Food Chem. Toxicol. 2011, 49, 1242–8.
- Soylak, M.; Balgunes, H. J. Hazard. Mater. 2008, 155, 595–600.
- López-García, I.; Viñas, P. et al. Talanta 2009, 78, 1458.
- Maranhão, T.A.; Martendal, E. et al. Spectrochim. Acta Part B 2007, 62, 1019.
- Zhao, L.; Zhong, S. et al. J. Hazard. Mater. 2012, 239–40, 206–12.
- Ghaedi, M.; Shokrollahi, A. et al. J. Hazard. Mater. 2012, 168, 1022–7.
- Sato, N.; Mori, M. et al. Talanta 2013, 117, 376–81.
- Burguera, J.L.; Burguera, M. Spectrochim. Acta Part B 2009, 64, 451.
- Jain, V.K.; Mandalia, H.C. et al. Talanta 2009, 79, 1331.
- Christou, C.K.; Anthemidis, A.N. Talanta 2009, 78, 144.
- Dakova, I.; Karadjova, I. Talanta 2009, 78, 523.
- Dai, B.; Cao, M. et al. J. Hazard. Mater. 2012, 219–20, 103–10.
- Zhang, D.; Su, H.D. et al. Spectrosc. Spect.Anal. 2008, 28, 218.
- Zhang, D.; Hou, P. Acta Chim. Sinica 2009, 67, 1336.
- Zhang, D.; Zhang, W.-J. et al. Spectrosc. Spect. Anal. 2009, 29, 693.
- Zhang, D.; Yuan, Z.-G. et al. Rare Metal Mater. Eng. 2009, 38, 2207.
- Zhang, D.; Yu, P. et al. J. AOAC Int. 2011, 93, 1925.
- Zhang, D.; Zhang, C.L. et al. J. Hazard. Mater. 2011, 186, 971–7.
- Wang, M.; Zhang, D. et al. J. Chin. Ceramic Society 2010, 38(2), 305–9.
The authors are with the School of Environmental and Chemical Engineering, Shenyang Ligong University, Shenyang 110159 P.R. China; tel.: +86 24 24680345; fax: +86 24 24682220; e-mail: [email protected].