Cancer research is as necessary as ever, especially if we are to fulfill the promise of personalized (or “precision”) medicine based on specific cancer therapies directed to known molecular targets. Fortunately, discovery is possible at a faster and more efficient pace with the help of innovative new research techniques.
Every year, roughly 14 million people learn they have cancer, and eight million people die from the disease, according to global statistics from the Centers for Disease Control and Prevention. However, research suggests that one-third of cancer deaths can be prevented with the right tools and education.
Droplet digital PCR
One emerging tool is a pioneering technology called droplet digital PCR™ (ddPCR™) (Bio-Rad Laboratories, Pleasanton, CA). In the past year and a half, a growing number of cancer researchers have begun using ddPCR for its ability to interrogate the cancer genome with unprecedented levels of sensitivity and precision. Although it is not the first implementation of digital PCR (dPCR), its unparalleled performance, quick turnaround time, and affordability are causing scientists to increasingly recognize its potential as a tool in the detection and monitoring of cancer, which can ultimately save lives.
The power of partitioning—precision, sensitivity, and robustness
First, let’s look closer at what dPCR entails and why it is advantageous for researchers today. At its core, dPCR is a method of counting nucleic acid molecules. It works by partitioning the sample in such a way that either zero or only a small number of nucleic acid molecules of interest (i.e., targets) are present in each partition.
This partitioning happens by dividing the sample into compartments (or partitions)—either microfluidic chambers or droplets, though historically, PCR-plate wells were used. The sample is then amplified to endpoint using normal PCR conditions (in a conventional thermocycler for ddPCR). An absolute measurement of the target concentration is achieved by scoring the positively and negatively fluorescent partitions. Unlike quantitative real-time PCR (qPCR), dPCR eliminates the need for a standard curve.
Eliminating the standard curve reduces error and improves precision in ddPCR. This enhanced precision enables day-to-day and lab-to-lab reproducibility, a long-standing weakness of prior methods. In addition, because samples are partitioned, the impact of competing background DNA is reduced, allowing for greater discrimination between similar sequences. In addition, because reactions are run to endpoint, they are more tolerant of potential sample inhibitors.
Introduced in 2011, the QX200 Droplet Digital PCR (ddPCR) system from Bio-Rad has become the most widely adopted approach to dPCR—with peer-reviewed publications describing use of the technology now appearing at a rate of about one every three days. It offers greater ease-of-use, enhanced performance, higher throughput, and lower costs compared to previous dPCR implementations, such as fluidic chip-based methods. The ddPCR system partitions samples into 20,000 droplets per well (in a 96-well plate), enough to give sufficient single-well precision and sensitivity for most high-throughput applications (300 samples/ day), but with the flexibility to merge sample wells in silico when still greater precision or sensitivity is desired.
Empowering cancer research
dPCR is being used in basic research, translational research, and clinical cancer research. In basic research, ddPCR allows researchers to examine tumor heterogeneity, clonal evolution, and metastasis, and to answer cell origin questions. In translational and clinical settings, ddPCR is being used to monitor drug resistance, identify alternative druggable targets, and prevent overtreatment, as well as measure dose-responses in targeted therapy underlying personalized medicine treatments.
Rare cancer mutation detection is one of the most common applications for ddPCR—used to test prognostic and predictive indicators, monitor residual disease, and preselect patients for clinical trials. Copy number variation (CNV) identification is another common ddPCR application. CNVs are structurally variant regions that involve either gains or losses of genomic DNA that have been shown to be associated with cancer.
The level of sensitivity offered by the Bio-Rad ddPCR system is also helping researchers quantify cancer biomarkers. Thus, ddPCR could be the key to bringing liquid biopsies—one of medicine’s holy grails—closer to clinical reality. These tests use blood or other body fluids such as urine instead of tissue from traditional biopsies to detect cancer, track its progress, and guide treatment decisions. ddPCR is able to isolate one molecule of mutant DNA into a droplet with just a few corresponding wild-type molecules, thus making the mutant DNA more detectable. What was previously undetectable with other methods can now be quantified.
The following are real-world examples of ddPCR’s impact across the full spectrum of cancer research from bench to bedside.
A starting point for personalized medicine
The ability to measure genomic amplifications of oncogenes is critical to developing targeted cancer therapies and demands high precision. However, generating accurate copy number (CN) measurements from small amounts of poor-quality DNA found in formalin fixed paraffin embedded (FFPE) tumor samples—already a mix of normal and tumor cells—makes the task even more challenging.
Traditionally, scientists have used qPCR and microarray-based assays to quantify these FFPE cancer tissue samples, but these methods are limited because they inherently lack the necessary precision as well as the tolerance to degraded FFPE DNA and its associated inhibitors. In contrast, the inherent measurement precision of ddPCR is more robust to the suboptimal PCR conditions afforded by FFPE samples.
In a recent study,1 researchers in the Ji Research Group at the Stanford University School of Medicine demonstrated that ddPCR is superior to qPCR for DNA copy number analysis of archival material. Using ddPCR, they measured a sevenfold amplification of the FGFR2 locus in an FFPE-processed gastric tumor, which was very similar to the value determined using a microarray analysis on a “matched” flash-frozen sample. Analysis of the same FFPE tumor sample with qPCR found a drastically different copy number. These results show that ddPCR is more accurate than qPCR for determining copy number variants in FFPE-derived samples.
The next frontier: microRNAs as cancer biomarkers
MicroRNAs (miRNAs) regulate the expression of thousands of genes in both normal and pathophysiological processes, including cancer, making them an intensive area of biological research. Numerous studies have shown that these small, noncoding RNAs have great potential as biomarkers, as researchers can monitor them in samples from tissues, cells, and body fluids—such as urine, cerebrospinal fluid, blood, plasma, serum, and sputum.
qPCR has traditionally been used to measure miRNAs; however, the high interlaboratory and interday variability of measurements in serum or plasma undermines their use as reliable blood-based biomarkers.
In a study2 published in Nature Methods, Dr. Muneesh Tewari, a researcher at the University of Michigan, evaluated the diagnostic potential of miRNA as an early biomarker for prostate cancer. The study showed that ddPCR increases reproducibility of the miRNA quantification process, making it feasible for results to be compared at different times. When they tested ddPCR versus qPCR on cDNA from six different synthetic miRNAs, in both water and serum, on three separate days, they found that ddPCR demonstrated greater precision—48 to 72% lower coefficients of variation—with respect to qPCR-specific variation.
They also found that ddPCR improved day-to-day reproducibility sevenfold relative to qPCR and, importantly, ddPCR enabled better discrimination of cancer samples from controls based on the level of a single miRNA.
The team’s ultimate goal is to develop biofluid-based approaches for disease detection and monitoring with simplicity and reproducibility in mind.
T-lymphocyte levels predicting patient outcomes in ovarian cancer
Tumor-infiltrating T-lymphocytes (TILs) are a type of tumor-attacking immune cell that is strongly associated with improved cancer survival. Clinicians would like to use TIL counts in tumors to predict survival outcomes and determine which patients may benefit from targeted immunotherapies. However, current immunohistochemical methods for scoring their abundance are too subjective and variable between labs to allow for their rigorous evaluation as a prognostic factor.
Dr. Jason Bielas, Associate Member of the Public Health Sciences and Human Biology Divisions at the Fred Hutchinson Cancer Research Center, found that ddPCR has the ability to comprehensively quantify the number of TILs in a tumor based on the shared genomic signature on the surface of each TIL.3
Researchers first demonstrated that their QuanTILfy assay can be used to accurately and reproducibly characterize T-cell clonality in patients with T-cell acute lymphoblastic leukemia—results that they validated using deep sequencing.
The team then demonstrated that the assay could robustly quantify the abundance of TILs in ovarian cancer from different patients, as well as spatial differences in TIL numbers within a single tumor. Finally, they performed the assay on primary tumor samples from 30 ovarian carcinoma patients with known survival outcomes. They found that TIL frequency was approximately threefold higher in those who survived more than five years as compared with those who survived less than two years, showing that TIL levels correlate positively with patient survival.
The robustness of the ddPCR-based QuanTILfy assay may surmount the limitations of existing immunohistochemical methods for TIL enumeration and enable this biomarker to be developed for prognosis and treatment of ovarian and other cancers.
Quantification of plasma cfDNA by liquid biopsy in a melanoma patient
An increasing number of translational studies are finding that ddPCR is well-suited for investigating the use of liquid biopsy in patient treatment and monitoring (see sidebar). In a recent illustrative study in Cancer Discovery,4 researchers described a patient with metastatic BRAF-mutant melanoma. Treatment with vemurafenib led to a dramatic shrinkage of his melanoma, but it induced proliferation of a chronic myelomonocytic leukemia (CMML) due to a previously unrecognized NRAS mutation. The researchers hypothesized that treating the patient with combined therapy of RAF and MEK inhibitors would treat the melanoma and reduce proliferation of the patient’s CMML, respectively.
Liquid biopsy literature
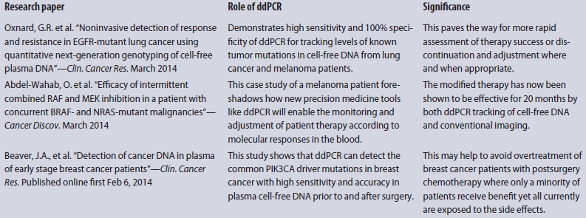
Based on white blood cell counts and radiographic imaging, they were able to arrive at an effective treatment regimen that beat back both cancers with less toxicity. During 20 months of evolving and improved treatment, ddPCR was used in parallel to quantify the melanoma-derived (BRAFV600K) and CMML-derived (NRASG12R) DNA in the patient’s plasma. The close agreement of ddPCR results with conventional radiographic and hematological monitoring supported the future use of ddPCR monitoring as an alternative to frequent radiographic monitoring of melanoma.
It is a great example of personalized medicine at work and shows how ddPCR might be used in concert with traditional tools to optimize cancer treatment in real time.
References
- Nadauld, L.; Regan, J.F. et al. Quantitative and sensitive detection of cancer genome amplifications from formalin fixed paraffin embedded tumors with droplet digital PCR. Transl. Med. Sept 2012, 2(2).
- Hindson, C.M.; Chevillet, J.R. et al. Absolute quantification by droplet digital PCR versus analog real-time PCR. Nature Methods Sept 2013, 10, 1003–5.
- Robins, H.S.; Ericson, N.G. et al. Digital genomic quantification of tumor-infiltrating lymphocytes. Sci. Transl. Med. Dec 2013, 5(214), 214.
- Abdel-Wahab, O.; Klimek, V.M. et al. Efficacy of intermittent combined RAF and MEK inhibition in a patient with concurrent BRAF and NRAS mutant malignancies. Cancer Discovery March 2014.
Dr. George Karlin-Neumann is Director of Scientific Affairs at Bio-Rad’s Digital Biology Center, 2000 Alfred Nobel Dr., Hercules, CA 94547, U.S.A.; tel.: 510-741-1000; e-mail: [email protected]; www.bio-rad.com