Most cellular structures and organelles involved in physiologically important processes are too small to be resolved by optical microscopes. Conventional scanning electron microscopes (SEMs) do not perform well on low-contrast, light-element materials, and the harsh preparation techniques required easily destroy or distort delicate biological structures. The Magellan™ extreme-resolution SEM (FEI Company, Eindhoven, The Netherlands) can resolve nanometer-scale detail on biological specimens with minimal artifacts from beam damage or sample preparation. The key to these capabilities is the microscope’s ability to achieve subnanometer resolution at very low beam energies that reduce beam penetration and minimize sample damage. The fully integrated work flow from sample preparation to imaging boosts laboratory productivity and expedites discovery.
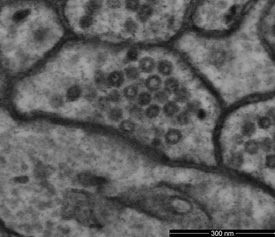
Figure 1 - Bilayer structure of membrane (less than 5 nm in width) resolved in image of the surface of a bulk specimen using the low-energy directional backscattered electron detector. (Sample courtesy of Dr. M. Ellisman, University of California San Diego.)
The very low voltage performance is made possible by the use of a monochromator to reduce the energy spread of the electron beam. By reducing the effects of chromatic aberration, it permits subnanometer beam diameters at accelerating voltages down to well below 1 kV. At these voltages, beam penetration is also dramatically reduced, resulting in very high image resolution even on biological samples (Figure 1).
This benefit is further enhanced by beam deceleration technology, which applies a negative bias to the sample, slowing beam electrons and further reducing their landing energy and penetration depth. In a typical case, an accelerating voltage in the column of 2 kV may be opposed by a decelerating voltage of –1.5 kV on the sample, for a net landing energy of 500 eV. The decelerating field also acts as an additional lens that reduces beam diameter by 10–50%.
Building contrast and reducing noise
Although a small beam diameter is desirable, it is useless if it does not contain sufficient current to generate adequate imaging signal levels. Resolution is not the sole determinant of image quality. Contrast and low noise are also required, and fundamentally related. The more signal collected, the greater the number of statistically significant gray levels the image can present. As the signal accumulates, random variations in the signal average out spatially and become less significant at any one point. “Adequate” signal levels might be best specified as a level sufficient to yield the required precision in the data (which translates into contrast and low noise in the image) over an acceptable acquisition time. That time might be determined by the convenience of the operator or by some limit imposed by degradation of the sample. A common limit in biological applications is the sample’s tolerance for exposure to the electron beam.
Important factors are the current available in the beam and the efficiency with which the imaging signal is collected and measured. The amount of current available is determined largely by the type of electron source, in particular by a parameter known as its brightness, which measures its angular intensity. The brighter the source, the more current that can be focused into a beam of a given diameter. The brightest are field emission sources, which can be either cold field emission (CFE) or superior Schottky (thermally assisted) field emission.
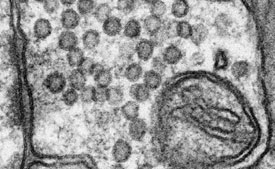
Figure 2 - Scanning transmission electron microscope image of mouse cerebellum resolves bilayer structure of membrane with low electron dose of 1200 electrons per nm2. (Sample courtesy of Graham Knott, École Polytechnique Fédérale de Lausanne [EPFL], Lausanne, Switzerland.)
Also important is the efficiency of the detectors. Different signals provide different information about the sample. Each detector is optimized for a particular energy range and emittance angle of the emitted electrons. However, it is generally true that the higher the detection efficiency, the better. This is particularly true with delicate biological samples, where, in order to avoid damage to the sample, the goal is often to use the lowest possible beam current that still generates adequate signal levels (Figure 2). Lower beam energies also reduce beam damage.
Finding features with light microscopy
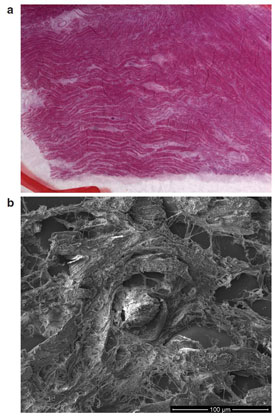
Figure 3 - Correlation of a) light microscopy with b) electron microscopy.
Correlative SEM is an exciting new field that allows researchers to quickly locate features identified with light microscopy techniques, such as fluorescent tags, for high-resolution examination in the SEM. An electronic representation of the light microscope image is imported into the SEM. The operator registers features that are recognizable in both images. After registration, the SEM will automatically navigate to any location designated by the operator in the light image.
A histology sample (Figure 3) is examined using light microscopy, and foreign particles that might contribute to the cause of death are identified. The position of the particles is transferred to the SEM and the stage is automatically driven to the exact location of the particles for viewing under high magnification. Energy-dispersive spectroscopy is performed for elemental analysis.
Preparing samples
Cryo sample preparation allows researchers to investigate biological samples in their natural, fully hydrated state. In many biological specimens, water is essential to the structural integrity of the sample. However, liquid water is incompatible with the vacuum levels required for high-resolution, low-voltage microscopy. Conventional sample preparation techniques use a variety of chemical and physical procedures to remove water and replace it with a vacuum-tolerant material. These procedures can easily distort delicate biological structures and introduce artifacts into the final image. Cryo techniques seek to avoid much of this risk by freezing the water in place. When the sample is small enough, the freezing process can be so fast that the water does not have time to crystallize, a process called vitrification, allowing the preservation of even the most delicate structure. High-pressure freezing will furthur improve the quality of the preparation, particularly for larger specimens.
Cryo sample preparation time is generally very short due to the simple processes employed. The sample is mounted on the transfer device, then plunge-frozen in the cryogenic unit. It is then transferred under vacuum in a low-temperature state to the preparation chamber of the cryo system. Here it can be sublimed or fractured and sputter coated. Finally, it is transferred at a known temperature to the specimen chamber of the SEM for observation.
Samples that show extensive water-containing structures on the surface such as plant leaves and petals can be cryogenically frozen to retain the structures intact. Plunge-freezing with liquid nitrogen is sufficient for this type of sample, since faster freezing with high pressure will not improve surface preservation due to the bulk of the sample and the thick membranes within.
Cryo fracture is a technique whereby the sample is frozen and fractured at a specified temperature. The nature of the fracture can be determined by the temperature, making the ability to achieve and hold the specified temperature a critical capability. Cryo fracturing exposes internal structure and provides an opportunity to relate it to surface features (Figure 4).
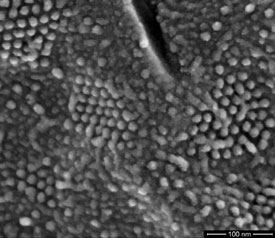
Figure 4 - Transmembrane particles (in hexagonal arrays) clearly resolved in a freeze-fractured preparation of yeast cell nuclear membrane (cryo-SEM beam deceleration at a landing energy of 500 eV). (Figure courtesy of Dr. A. van Aelst, Wageningen Electron Microscopy Centre [WEMC], Wageningen, The Netherlands.)
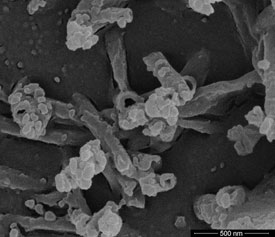
Figure 5 - Image of pine needle wax.
The internal structure of cooled specimens can be revealed by freeze fracture. Even greater detail can often be revealed by carefully raising the temperature of a freeze-fractured hydrated specimen to a point at which water begins to sublime at a controlled rate (–90 to –100 °C). This etching process can be arrested again by lowering the temperature (Figure 5).
Stabilizing low-melting-point solids
Suspensions, oils, fats, waxes, plastic polymers, and emulsified products are often either damaged by the electron beam or not stable in the vacuum environment of an SEM. Such samples can be cryo stabilized by freezing and transferring to a cryo preparation chamber under vacuum. There they can be fractured, sputter coated with a conductive layer, and examined on a cold stage in the SEM. Fracturing such samples gives information on the distribution and size of the various phases and components within an individual formulation or product. Since specimen preparation and examination can be completed within a short time, productivity is an added bonus.
The sample can be completely dehydrated if it is left long enough at a temperature slightly higher than that of the decontaminator.
Conclusion
The Magellan SEM provides researchers in the life sciences with real, usable, nanometer-scale resolution on biological samples in their natural, fully hydrated state. Its combination of low energy spread, beam deceleration, high-efficiency detectors, correlative navigation, and optional cryo handling delivers very high image quality and resolution, and can provide a streamlined imaging work flow specifically designed for life science research.
Mr. Lich is Cell Biology Marketing Manager, FEI Company, Achtseweg Noord 5, Building AAE, 5651 GG Eindhoven, The Netherlands; tel.: +31 40 23 56709; e-mail: [email protected].