As first described in Ref. 1, an alternative liquid–liquid extraction technique was originally conceived, born, and cultivated in an environmental lab setting to enable holding time obligations to be more easily met. Many studies have been conducted since to perfect the technique, examine its reliability, and explore its applicability. The method, called bottle liquid–liquid extraction (BLLE, i.e., “Billie”) ultimately simplifies and reduces the cost of extracting organic chemicals from water. It is a green alternative to separatory funnel (SF) and continuous liquid–liquid extraction (CLLE) techniques commonly in use in most environmental and other laboratories.
The procedure enables organic compounds to be extracted directly from water contained within original sampling containers (glass bottles). The volume of solvent required is sharply reduced, and holding times are more easily achieved while quality is maintained or improved. After a small amount of sample water is removed to make room for the addition of extracting solvent(s) and, in some instances, salt, the bottles are subjected to a gentle (3 rpm) rotation for up to an 18-hr period. The extracting solvent is removed from the bottle using a customized funnel (designed for this application) and concentrated using conventional techniques.
Since publication of the original paper, a convenient means to remove the extraction solvent from the bottles following the extraction process has been developed. A new device (funnel cock), designed and fabricated specifically for this application, is introduced in this supplemental paper.
Further studies were suggested to evaluate and optimize the many variables inherent in the procedure to yield additional reductions of solvent, labor, and cost. All equipment needed to perform the procedure is economical, reusable, rugged, and is now readily available.
Figure 1 – Photograph of funnel cock.Funnel cock and inversion chair
A major obstacle to the implementation of this method had been the lack of availability of a bottle attachment to effect the removal of solvent from the water bottle following the extracting process. In the studies conducted to develop this procedure, the entire contents of the bottles had to be transferred into a separatory funnel to facilitate the phase separation. This was effective but counter-productive to a goal of reducing the labor and complexity of the process. A mechanism (a funnel adaptation) as well as a holder to couple an inverted bottle to the mechanism was conceived and subjected to ruggedness testing. The funnel cock (Figure 1) is constructed to mate with two of the most common environmental water sample bottles, 1-L Boston round and 2-in.-diam wide-mouth bottles.
Following the bottle rotation extraction, instead of transferring the bottle contents into a separatory funnel, the bottle cap is removed and the bottle inverted onto the funnel cock housed in a holder (inversion chair) (Figure 2). The funnel cocks are internally scored to prevent a seal from forming between the internal wall of the funnel and the lip of the bottle. As the bottle and liquid contents are inverted, liquid overflow is retained in the upper part of the funnel and the heavier-than-water extracting fluid is able to be drained. A vacuum instantly forms in the bottle as it is overturned, restricting overflow to a cavity in the upper third of the funnel. As the stopcock of the funnel is opened, air enters the bottle about the etched surface at the lip of the bottle, enabling the heavier liquid layer to be discharged in a controlled manner.
Figure 2 – Photograph of inversion chair with inverted bottle and funnel cock.Just as with a separatory funnel, the funnel cock is made of a translucent material so that the interface between the liquid layers may be observed as it traverses the funnel. The construction material is also inert and durable to promote nearly infinite reuse.
Further information regarding the current availability of these items may be obtained at www.blle.org. A patent for the funnel cock has been applied for.
Experimental
As noted above, numerous experiments were conducted to optimize this procedure, to test the new devices, and to extend applicability to real-world samples and other methods. The original paper1 provided environmental analysis sample preparation procedures for acid and base neutral extractable semivolatile organics and for herbicides. Results were presented that were obtained extracting a laboratory control matrix (deionized/charcoal filtered water). The results for two more studies are presented here, examining the extraction of low-level pesticides and semivolatile organics from a challenging, real-world surface water—Sand Run Creek water, a tributary of the Cuyahoga River in Northeast Ohio.
Pesticide extraction (BLLE procedure for U.S. EPA Methods 608 or 8081)
Table 1 summarizes the culmination of dozens of earlier studies extracting organochlorine pesticides from deionized/charcoal filtered water. The particular experiment shown here reduces solvent volumes to practical minimums while examining the effect of salt. Earlier studies identified methylene chloride, diethyl ether, and sodium chloride as important and interactive players in the process. Sodium chloride significantly reduces the solubility of the solvents in the water, allowing less to be used. Ether had been shown to significantly improve recovery of a number of analytes over the use of methylene chloride alone. Even a very subtle effect of adding the heavier-than-water methylene chloride and lighter-than-water ether separately instead of premixing was contemplated and examined.
TABLE 1. PESTICIDE TECHNIQUE COMPARISON - BOTTLE LIQUID-LIQUID EXTRACTION SEPARATE SOLVENTS vs. SOLVENT MIX and THE EFFECT OF SALT
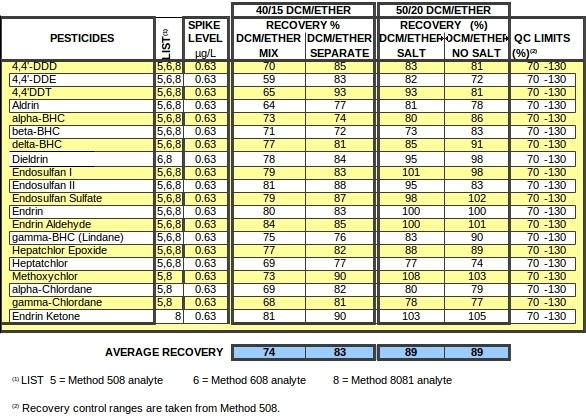
In this study, as indicated in Table 1, a combined total of up to 70 mL of solvent was added to 800 or 850 mL of water in amber Boston round liter bottles; 100 g of sodium chloride was also added to most of the bottles. The bottles were tumbled horizontally, overnight, at 3 rpm. To most bottles, methylene chloride and diethyl ether were added separately—DCM first settling to the bottom and ether remaining at the top. Overnight, as a consequence of the extracting process, the ether and methylene chloride combined completely to form a slightly buoyant but heavier-than-water mix. The funnel cock was used to discharge the solvent from the bottle for subsequent concentration and analysis. All experiments were conducted in duplicate to substantiate observations.
As discerned from Table 1, adding the methylene chloride and ether separately is markedly advantageous, whereas the need to add salt is equivocal. For most intents and purposes, with great ease and minimal cost, pesticides may be extracted efficiently overnight using just 50 mL of methylene chloride and 20 mL of diethyl ether.
As gleaned from this and other studies, the following general procedure is optimal for the extraction of neutral semivolatile organics (pesticides, petroleum hydrocarbons [DRO, ORO], polynuclear aromatic hydrocarbons [PAHs], etc).
Procedure (D)—neutral semivolatile organics:
Step 1) Remove sample bottle from refrigerated storage and allow to equilibrate to room temperature.
2) Shake bottle briefly to homogenize the sample. Discard up to 150 mL and mark water level for later measurement (note the volume of many Boston round bottles is 1050 mL, leaving an extraction volume of 900 mL).
3) Fortify sample with surrogate and batch QC samples with spike solutions prepared in water-soluble solvents as appropriate. Cap and invert once or twice to mix.
4) Add 50 mL of methylene chloride first (sinks to bottom); then float 20 mL of diethyl ether on top.
5) Cap bottle, place horizontally on rotation rack, and spin overnight (18 hr) at 3 rpm.
6) Remove bottle cap and invert bottle using the chair clasping the bottle to a funnel cock (stopcock closed). Gently tap the invented bottle with the palm of the hand or other implement to ensure that 100% of the solvent (methylene chloride/ether mix) settles into the funnel cock (note that approx. 20 mL of the original 70 will have solubilized into the water, leaving the recovery of just 50 mL).
7) Discharge the slightly buoyant but heavier-than-water solvent layer into an appropriately sized container (a 60-mL VOA vial is ideal).
8) Dry extract by adding a few grams of sodium sulfate directly to the 50-mL extract in the receiving vessel or by passing the extract through a bed of sodium sulfate.
9) Concentrate by conventional means and submit for analysis.
Semivolatile extraction (BLLE procedure for U.S. EPA Methods 625 or 8270)
Table 2 is the capstone of hundreds of semivolatile extractions extending the procedure to a real-world sample—a brown-tinged surface water obtained from a nearby creek.
TABLE 2. SEMIVOLATILE TECHNIQUE COMPARISON - BOTTLE LIQUID-LIQUID EXTRACTION OF SURFACE WATER SOLVENT AND SALT MINIMIZATION
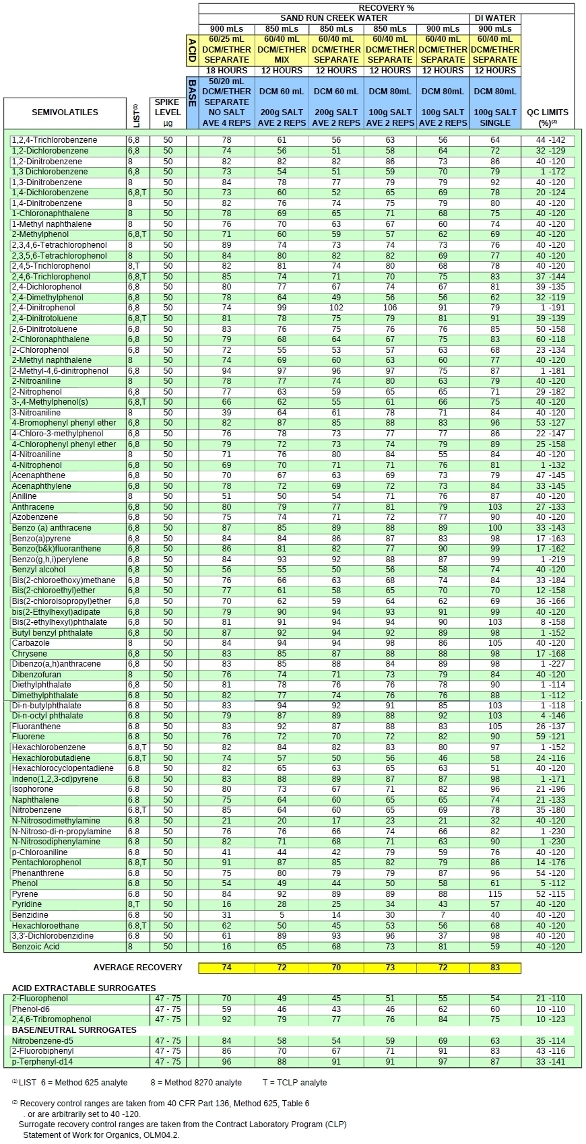
BLLE experiments were designed fortifying 900–850 mL of brown-tinged creek water collected in 1-L Boston round bottles. A deionized/charcoal filtered water sample was also analyzed as a control. Each was spiked with a 79-component mixture of semivolatile organics (Mega-Mix catalog nos. 31850, 31688, and benzoic acid (Restek, Bellefonte, PA) containing acid, neutral, and base extractables at a level of 50 μg for each of the 79 compounds. The six traditional 8270C surrogates were also added. The pH was first brought down to <2 (acid first extraction), and up to 60 mL DCM and 40 mL diethyl ether were added as a mix or separately as indicated in the header of Table 2. Following a 12- or 18-hr turn at 3 rpm, the bottle was inverted with the chair holder onto a funnel cock. The heavier-than-water solvent was discharged into a 250-mL bottle. Sodium chloride was then added to the water retained in the bottle for most experiments and the pH was readjusted to >12 (base neutral extraction); 60–80 mL of solvent was utilized for a second 18–12 hr turn at 3 rpm. The solvent from this second extraction was combined with the original acid neutral extract retained in the 250-mL bottle. The combined acid/neutral and basic extracts were concentrated to 1-mL using a Turbovap apparatus (Zymark, Hopkinton, MA) prior to analysis by conventional environmental 8270C/625 methodology.
Nearly all the components were recovered with greater than 75% recovery in all experiments. Although these experiments were conducted over a two-month period and the creek water content likely changed with the separate sampling events, important subtleties are apparent. Among the analytes identified as problematic in the original paper, 3-nitroaniline, pyridine, and benzoic acid clearly benefit from the addition of salt during the base extraction. The real-world matrix appears to account for about an overall 10% suppression of recovery. The results obtained for the benzidines are erratic and difficult to gauge. Given that the makeup of the surface water collected (particularly the dissolved solids content) was likely different for each experiment, this is not surprising.
Based on this and numerous prior studies, the following general procedure is advocated for the extraction of base/neutral/acid (BNA) semivolatile organics:
Procedure (E)—BNA semivolatile organics:
Step 1) Remove sample bottle from refrigerated storage and allow to equilibrate to room temperature.
2) Shake bottle briefly to homogenize the sample. Discard some of the sample so that a minimum volume of 150 mL is vacated to allow for subsequent addition of solvents and reagents (in the case of 1050-mL Boston round bottles, 900 mL of water may be retained). Mark water level on the bottle for later measurement.
3) Fortify sample with surrogates and QC samples with appropriate solutions.
4) Adjust sample pH with approx. 3 mL of 12N sulfuric acid. Check that pH is ≤2 with wide-range pH paper.
5) Add 60 mL of DCM followed by 40 mL of (ethanol-preserved recommended) diethyl ether (DCM on bottom; ether on top of the water). (Be mindful to maintain the accuracy of the DCM to ether ratio—too much ether will cause the ultimate solvent layer to be adversely buoyant).
6) Cap bottle; place horizontally on rotation rack and spin for 12 hr at 3 rpm.
7) Uncap and place bottle onto an inversion chair and invert onto appropriately sized funnel cock. Discharge solvent layer (heavier than water) into a 250-mL bottle. Add 10 g sodium sulfate to bottle to commence drying the extract).
8) Add 100 g sodium chloride to remaining water and shake to dissolve. Readjust pH of the water to ≥12 (check with pH paper) using approx. 9 mL 6N NaOH.
9) Add 80 mL of DCM and return to rotisserie for a second 12-hr period.
10) Uncap and invert once more onto funnel cock using the inversion chair. Combine DCM layer with initial DCM/ether extract.
11) If sodium sulfate was added to the extract bottle, allow extract to remain in contact with sodium sulfate for at least 2 hr, mixing periodically. If the sodium sulfate forms clumps, add more sodium sulfate until it remains free flowing. (Adding sodium sulfate directly to the extract in place of a common practice of pouring the extract through a funnel or column of sodium sulfate serves several purposes. The sodium sulfate remains in contact with the extract for longer periods of time, the successful removal of water is indicated by an absence of clumps, and much less sodium sulfate is required.)
12) Drain extract through a glass fiber filter and concentrate the extract using conventional evaporative techniques. Submit for analysis.
13) Recharge original bottle with tap water to the original mark and pour into 1000-mL volumetric flask to record exact volume extracted.
Results and discussion
The tables in this and the original paper summarize some of the variations in operating parameters that have been studied. The effect of different solvents, amount of solvent(s), extraction times, sample volumes, bottle size, addition of and amount of salt, rotation speed, pH adjustments, extraction temperature, dissolved solid content, and a host of other variables have been studied. Even a subtle effect of adding lighter- and heavier-than-water extraction solvents (ether and methylene chloride) separately as opposed to premixing them appears to have an impact on the process. The best results obtained to date in terms of speed, cost minimization, and high recovery are presented in this update. It is possible that the technique may be improved further through additional experimentation.
At the current state of development, Table 3 compares advantages and disadvantages of BLLE to the two most common liquid–liquid extraction techniques most commonly employed today.
TABLE 3. TECHNIQUE COMPARISONS SEPARATORY FUNNEL, CONTINUOUS LIQUID-LIQUID AND BOTTLE LIQUID-LIQUID EXTRACTIONS
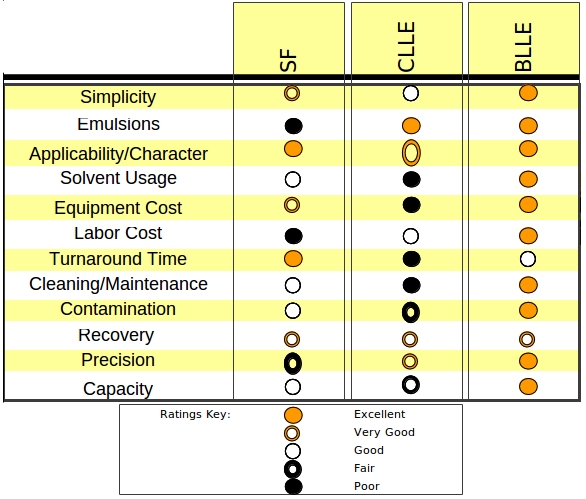
Original paper errata
Step 5 of the section detailing Procedure A improperly stated that “methanol-preserved” ether may be used. This should have read “ethanol-preserved.”
In Step 6 and elsewhere in the paper it was implied that bottles were spun at 12 rpm as opposed to the correct rate of 3 rpm. (The gear motor of the rotisserie actually turns at a rate of 12 to achieve a geared-down bottle turning rate of 3 rpm.) Step 9 of this same section should read “80 mL of 60/40” in place of “80 mL of 50/30.”
In the Results and Discussion section commenting on the performance of the compound 2-chloronaphthalene, it was incorrectly stated that U.S. EPA Method 625 calls for an “acid first” extraction. This should have read “base first.”
Reference
- Automated, Economical Sample Preparation: Bottle Liquid–Liquid Extraction. Am. Lab. 2009, 40(8),18–25; https://www.americanlaboratory.com/913-Technical-Articles/553-Automated-Economical-Sample-Preparation-Bottle-Liquid-Liquid-Extraction/.
Additional reading
- U.S. EPA Office of Solid Waste and Emergency Response, SW-846, 3rd ed., Rev 3; Dec 1996.
- Base/Neutrals and Acids. 40 Code of Fed. Reg., Part 136, Appendix A, Method 625; Office of the Federal Register, National Archives & Records Administration.
- Groundwater Monitoring List. 40 Code of Fed. Reg., Part 264, Appendix IX; Office of the Federal Register, National Archives & Records Administration.
Mr. Troost is with Summit Environmental Laboratories, 3310 Win St., Cuyahoga Falls, OH 44223,U.S.A.; tel.: 330-310-2150; e-mail: [email protected]; www.blle.org.