The precious metal palladium has been widely applied in the electronics, aerospace, chemical, metallurgy, automobile engines, and pharmaceutical industries due to its corrosion resistance, oxidation resistance, and excellent catalytic properties.1,2 In recent years, the development of analytical methods for the determination of trace amounts of precious metals in environmental water,3 geological,4 metallurgical and automotive catalyst converter samples,5,6 and biological materials7,8 has attracted considerable attention.
At present, determination of the presence of palladium is typically done by atomic absorption spectrometry (AAS),1,9–11 inductively coupled plasma-atomic emission spectrometry (ICP-AES), inductively coupled plasma-optical emission spectrometry ICP-OES), and inductively coupled plasma-mass spectrometry (ICP-MS).12–16 However, palladium is found in environmental waters and geological materials at trace and ultratrace levels, and its direct determination is beyond the scope of flame atomic absorption spectrometry (AAS). Therefore, a preconcentration procedure is often required.
Widely used techniques for preconcentration and separation include solvent extraction,17–20 ion exchange,21–23 co-precipitation,12 and solid-phase extraction (SPE).15,24–26 In recent years, SPE preconcentration and separation have become increasingly popular for the determination of trace levels of metals. The main SPE sorbents are chelating resin,27,28 modified barium-strontium titanate powder,29 and modified silica.30
With the development of nanomaterial science and technology, nanoparticulate metal oxides, such as TiO2 and ZnO, have been found to have very high adsorption capacity of heavy metal ions from environmental samples.31–33 For the first time, nanocalcium titanate powders have been used in the adsorption of heavy metals in water,32 and the results of these studies indicate that nanocalcium titanate powders have higher adsorption capacity than nanoparticulate metal oxides. However, nanocalcium titanate particles are so small that, when used in the adsorption of metal ions, they easily coacervate and lose activity and are difficult to recover. In order to correct these problems, nanocalcium titanate was fixed onto a glass fiber filter and used the adsorption of lead and cadmium ions in water with satisfactory results.34 However, use of immobilized nanocalcium titanate on glass fiber filter in the preconcentration of palladium has not been studied.
In the present work, the adsorptive potential of porous calcium titanate-based coated glass fiber filter (GPCTO) for the preconcentration of trace Pd(II) was assessed using the batch adsorption method. A new method using GPCTO as SPE agent has been developed for the preconcentration of trace palladium ion in water and geological sample analyses by flame atomic absorption spectrophotometry (FAAS).
Experimental
Materials and methods
A WYX-9003A atomic absorption spectrometer (Shenyang Yi Tong Analytical Instrument Co., Ltd., China), equipped with a hollow cathode lamp for palladium, was used for palladium determination. The measurements were done in air–acetylene flame, and the operation conditions are summarized in Table 1.
Table 1 – FAAS operating conditions

The pH values were measured with a PHS-3C acidometer (Shanghai REX Instrument Factory, China) supplied with a combined electrode. Adsorption and desorption experiments were carried out in a bath shaker (Jintan Experiment Instrument Factory, Jiangsu, China).
GPCTO was prepared in the authors’ laboratory as described in Ref. 34. A stock solution (1 g/L) of Pd(II) was prepared by dissolving 0.1666 g of palladium dichloride (Sinopharm Chemical Reagent Co., Ltd., Shanghai, China) in 5 mL of aqua regia and dilution to 100 mL with water. Working standards were prepared daily by appropriate dilution of the stock solution with water. All chemicals were guaranteed reagent grade. The water in this study was double-quartz sub-boiling distilled water.
General procedure
Pd(II) solution was placed into a 150-mL (or 1000-mL) calibrated Erlenmeyer flask with plug. The pH was adjusted to 5–7 with hydrochloric acid or sodium hydroxide solution. After diluting to the mark of 50 mL (or other volume) with water, 0.1 g of GPCTO was added. The Erlenmeyer flask was covered and shaken for 5 min in the bath shaker at 200 r/min. After filtration, the concentration of Pd(II) in filtrate was determined by FAAS, and absorption capacity was calculated. The adsorbent was then washed with water to remove any unadsorbed Pd(II). The adsorbed Pd(II) was eluted with 5 mL of 0.5 mol/L HNO3 solution by shaking for 5 min. Concentrations of the Pd(II) in the eluents were determined by FAAS, and recovery was calculated.
Results and discussion
Effect of pH on adsorption
In order to study the effect of pH on adsorption, the pH was adjusted in the range of 1–9 using hydrochloric acid or sodium hydroxide solution, and the adsorption procedure described above was applied. The results are shown in the Figure 1. The adsorption percentage of GPCTO to Pd(II) was high and pH dependent. Increasing the pH resulted in an increase in adsorption capacity. The adsorption percentage of Pd(II) in the pH range of 5–9 was 100%. In order to avoid the precipitation of metal ions at high concentration, pH 5–7 was selected as the optimal condition.
Figure 1 – Effect of pH on adsorption.Effect of shaking time on adsorption
The adsorption capacity was determined at different shaking times to study the influence of contact time on the adsorption of Pd(II) onto GPCTO. The results are showed in Figure 2. It can be seen that the reaction of adsorption was very rapid, and equilibrium was reached at 1 min. The adsorption capacity of GPTCO to Pd(II) was 43.35 mg/g. To complete the reaction, the shaking time was 5 min.
Figure 2 – Effect of contact time on Pd(II) adsorption.Elution
Based on Figure 1, the adsorption percentages of Pd(II) on the GPCTO was zero when the pH value was 2 or lower. Therefore, the elution of Pd(II) retained in the GPTCO was studied by the addition of 5 mL of HNO3 at concentrations ranging from 0.001 mol/L to 1.0 mol/L. The results showed that desorption recovery increased with an increase in HNO3 concentration. When the concentration of HNO3 was higher than 0.1 mol/L, the recoveries were up to 98%. Thus, for sufficient elution, in this study, 5 mL of 0.5 mol/L HNO3 was chosen.
Table 2 – Preconcentration and recovery of palladium
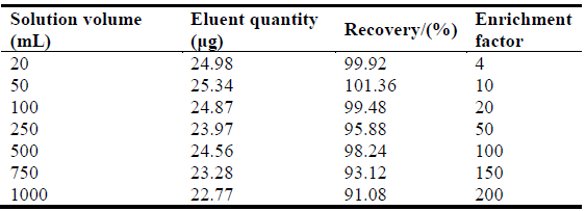
After elution, the GPCTO was dried to 105 oC and reused 10 times. Its adsorption performance did not decrease, indicating that this adsorption agent was very stable.
Enrichment factor and detection limit
To study the possibility of preconcentration, low concentrations of Pd(II) from large volumes—25.0 μg of Pd(II)—were dissolved in various volumes of water (i.e., 20 mL, 50 mL, 100 mL, 250 mL, 500 mL, 750 mL, and 1000 mL). After adsorption, GPCTO was eluted with 5 mL of elution liquid. The amounts recovered are listed in Table 2. It can be seen that when the solution volume was 1000 mL, the recovery was above 90%. The enrichment factor was 200. The detection limits (3× SD) were calculated using a procedural blank consisting of 200 mL water, 0.5 g GPCTO taken through the entire procedure as above, and calculated from 11 runs of the blank solution with concentrations of Pd(II) at 0.061 μg/L. The relative standard deviations (RSDs) for Pd(II) were 3.4% (n = 11, CPd = 10 μg/L).
Effect of interfering ions
The effects of common coexisting ions on the adsorption of Pd(II) were studied. Various interference ions were added to a 100-mL volumetric flask containing 10 μg of Pd(II). The results showed that recoveries of the Pd(II) remained in the 95–105% range, even in the presence of ions in the following concentrations: 1000 mg/L for Na+, K+, NH4+, and NO3; 500 mg/L for Ca2+, Mg2+, Al3+, and PO43–; 200 mg/L for Cr(III), Co2+, Cr(VI), and Cu2+; 100 mg/L for Zn2+, Ni2+, Fe3+, Au(III), and Pt(IV); and 10 mg/L for Pb2+ and Cd2+.
Application of the method
The above procedure was applied to well water, river water, and lake water. The well water sample was from Huishan of Shenyang and was analyzed immediately. The surface waters were collected from the Hun-he River and Nan-hu Lake (Shenyang, China). One hundred milliliters of water sample was preconcentrated using the optimized SPE technique after digestion with concentrated nitric acid. The results obtained and the associated recoveries are shown in Table 3.
Table 3 – Determination of palladium in water samples (n = 6)
aNot detected.
The proposed method was also applied to gold ore (from the mining companies of Liaoning) at optimal conditions. Each mine stone was ground, and a portion of it was weighed in the range of 4–6 g and digested in aqua regia by refluxing the mixture for 12 hr. The solution of each sample was filtered. The filtered solution was diluted with concentrated nitric acid up to 25 mL. Finally, 5.0 mL of this solution was poured into a volumetric flask; after adjusting the pH, the volume of solution was adjusted to 50 mL.
Table 4 – Determination of palladium in gold ore samples (n = 6)

The solution was then adsorbed and eluted using the proposed method at the optimal conditions. The blanks were prepared in the same way as the sample, but the sample was omitted. The analytical results and associated recoveries are given in Table 4. The recovery was 91.4%–97.7 %, which is excellent for trace analysis. Thus, the proposed method demonstrated the suitability of this sorbent for the preconcentration and determination of trace palladium.
Conclusion
The results show that Pd(II) can be quantitatively retained by GPCTO in the pH range of 5–7, with an adsorption time of 1 min. The adsorption capacity of GPTCO to Pd(II) was 43.35 mg/g. Pd(II) adsorbed on GPCTO can be completely eluted by using 5 mL, 0.5 mol/L HNO3. The enrichment factor was more than 200. The detection limit of this method for Pd(II) was 0.061 μg/L. The proposed method has been applied to the determination of trace amounts of palladium in water and gold ore with satisfactory results.
The authors are with the School of Environmental and Chemical Engineering, Shenyang Ligong University, Shenyang 110159 P.R. China; tel: +86 24 24680345; fax: +86 24 24682220; e-mail: [email protected].
References
- Tokalıoğlu, S.; Oymak, T. et al. Anal. Chim. Acta 2004, 511(2), 255–60.
- Zereini, F.; Alt, F. Alt, Eds. Palladium Emission in the Environment; Springer-Verlag: Berlin Heidelberg, 2006.
- Saçmacı, S.; Kartal, S. Talanta 2013,109, 26–30.
- Chakrapani, G.; Mahanta, P.L. et al. Talanta 2001, 53(6), 1139–47.
- Anthemidis, A.N.; Themelis, D.G. et al. Anal. Chim. Acta 2000, 412(1–2), 161–7.
- Anthemidis, A.N.; Themelis, D.G. et al. Talanta 2001, 54(1), 37–43.
- Vaezzadeh, M.; Shemirani, F. et al. Food Chem. Toxicol. 2010, 48(6), 1455–60.
- Fan, Z.; Jiang, Z. et al. Anal. Chim. Acta 2004, 510(1), 45–51.
- Liang, P.; Zhao, E. et al. Talanta 2009, 77(5), 1854–7.
- Sánchez Rojas, F.; Bosch Ojeda, C. et al. Talanta 2006, 70(5), 979–983.
- Ozturk, N.; Numan Bulut, V. et al. Desalination 2011, 270(1–3), 130–4.
- Petrova, P.; Velichkov, S. et al. Spectrochim. Acta Part B: Atomic Spectroscopy 2010, 65(2), 130–6.
- Wu, Y.; Jiang, Z. et al. Talanta 2004, 63(3), 585–92.
- Fang, J.; Liu, L.-W. et al. Spectrochim. Acta Part B: Atomic Spectroscopy 2006, 61(7), 864–9.
- Moldovan, M.; Milagros Gómez, M. et al. Anal. Chim. Acta 2003, 478(2), 209–17.
- Nakajima, J.; Ohno, M. et al. Talanta 2009, 79(4), 1050–4.
- Traeger, J.; König, J. et al. Hydrometallurgy 2012, 127–8, 30–38.
- Swain, B.; Jeong, J. et al. Hydrometallurgy 2010,104(1), 1–7.
- Igarashi, S.; Ide, N. et al. Anal. Chim. Acta 2000, 424(2), 263–9.
- Lopez Garcia, I.; Martinez Aviles, J. et al. Talanta 1986, 33(5), 411–14.
- Moawed, E.A. Anal. Chim. Acta 2006, 580(2), 263–70.
- Hubicki, Z.; Wołowicz, A. J. Hazardous Mater. 2009, 164(2–3), 1414–19.
- Kovacheva, P.; Djingova, R. Anal. Chim. Acta 2002, 464(1), 7–13.
- Godlewska-Żyłkiewicz, B.; Kozłowska, M. Anal. Chim. Acta 2005, 539(1–2), 61–7.
- Davudabadi Farahani, M.; Shemirani, F. et al. Talanta 2013, 109, 121–7.
- Kovalev, I.A.; Bogacheva, L.V. et al. Talanta 2000, 52(1), 39–50.
- Birinci, E.; Gülfen, M. et al. Hydrometallurgy 2009, 95(1–2), 15–1.
- Çetin, T.; Tokalıoğlu, S. et al. Talanta 2013, 105, 340–6.
- Zhang, D.; Yu, P. Asian J. Chemistry 2012, 24(4), 1715–18.
- Sharma, R.K.; Pandey, A. et al. J. Hazard. Mater. 2012, 209–10, 285–92.
- Zhang, D.; Su, H. et al. Spectrosc. Spect. Anal. 2008, 28(1), 218–21.
- Zhang, D.; Hou, P. Acta Chim. Sinica 2009, 67(12), 1336–42.
- Zhang, D.; Zhang, C. et al. J. Hazard. Mater. 2011, 186, 971.
- Zhang, D.; Ping, Yu et al. J. AOAC Int. 2011, 93(6), 1925–33.