Charge transfer chemical ionization (CI) was among the ionization types studied decades ago by researchers investigating fundamental characteristics of chemical ionization.1 However, the technique was not widely adopted for use in vacuum source mass spectrometry. This is likely due, at least in part, to practical difficulties associated with maintaining the proper reagent gas density to promote efficient charge exchange in a mass spectrometer equipped with an ion source under vacuum. Conversely, atmospheric pressure ionization sources have a very high gas capacity, allowing optimum conditions for charge exchange CI without placing excessive (gas) load upon the vacuum system of the mass spectrometer.2
More recently, an Atmospheric Pressure Gas Chromatography (APGC) system was developed by McEwen,3 then at DuPont (Wilmington, DE). The system couples a standard GC to a modified LC/MS instrument (Waters Corp., Milford, MA). The system was further developed and commercialized by Waters in 2008. The source is based on a Waters universal source architecture for which the sample cone used in electrospray is replaced with a specific ion chamber for APGC (Figure 1).
Figure 1 – Schematic of the APGC ionization/reaction chamber showing the orientation of components during GC/MS operation.A corona pin is present inside the ion chamber and is used to generate a nitrogen plasma that reacts with either the analyte molecules or any modifier present in the ionization region.
Mechanisms of ionization
There are two primary mechanisms of ionization that APGC can undergo:
- Charge transfer initiated by corona discharge ionization of the nitrogen in the source to generate radical cations of nitrogen that can then undergo charge transfer reactions with analyte molecules to generate radical cations of the analyte molecules. This form of ionization is favored by nonpolar compounds (see Figure 2).
- Protonation where the proton source can be a protic solvent such as water, MeOH, etc. This form of ionization is favored by relatively polar compounds (see Figure 3).
Figure 2 – Mechanism of ionization—charge transfer.
Figure 3 – Mechanism of ionization—proton transfer.It is possible to influence the ionization in APGC by altering source conditions. APGC ionization is “soft,” i.e., fragmentation of compounds is very low, especially when compared to electron ionization (EI). This means that sensitivity and selectivity are increased and multiple reaction monitoring (MRM) precursor selection is simplified. The system is designed such that it can be changed from LC to GC quickly without the need to break vacuum.
The use of APGC for the analysis of persistent organic pollutants (POPs) was investigated using a Waters Xevo TQ-S as an ultratrace quantification platform. Two examples of the data are given below.
Analysis of dibenzo-p-dioxins and furans
Kotz, Winterhalter, and Traag used a Xevo TQ-S for the analysis of dioxins and furans and compared it to existing GC-high-resolution MS (GC-HRMS). Sample preparation and cleanup were performed using a validated method developed by the European Union Reference Laboratory (EURL),4 and were identical for both techniques. A reduced cleanup was also analyzed to test instrument selectivity. Calibration solutions (0.0125–0.5 pg/μL, 2,3,7,8-TCDD [2,3,7,8-tetrachlorodibenzo-p-dioxin]) were analyzed to check the linearity of the system. Dilutions of the lowest standard (31.3, 12.5, 6.3, 3.1 fg, TCDD) were used to test the absolute sensitivity of the technique.
Results and discussion
Sensitivity
The raw, unsmoothed chromatograms for the MRM transition (m/z 320 > 257) for 2,3,7,8- TCDD (absolute concentrations between 2.5 pg and 3.1 fg) are shown in Figure 4. The peak areas showed good correlation to the absolute injected amount on column with an R2 > 0.99.
Figure 4 – Correlation of peak areas and concentrations for 2,3,7,8-TCDD.Calculation of the limit of quantification (LOQ)
Data acquired using APGC-MS/MS show an appreciable level of background noise; this permits the use of signal-to-noise to calculate limits of detection. However, in order to check the reliability of the results in this low concentration range, the ion abundance ratio and the relative response factors are important additional criteria. The deviations of the theoretical ion abundance ratio should be within ±15% of theoretical value and the deviation of the relative response factor of the mean value ≤30% (with a CV ≤20% for the complete calibration). Applying these criteria, LOQs were calculated based on calibration standards and dilutions. For 2,3,7,8-TCDD and 2,3,7,8-TCDF (2,3,7,8-tetrachlorodibenzo-p-furan), LOQs were obtained in the range of 10–30 fg on column. These results are similar (and in some cases better) than a magnetic sector instrument.
Results for sample extracts
Results of APGC-MS/MS measurements were compared with assigned values from proficiency testing (PT) and mean values of quality control materials obtained from GC-HRMS analysis. The APGC-MS/MS system showed sufficient sensitivity to analyze samples in the range of action and maximum levels. Deviations of the results of APGC-MS/MS from reference values were below 20% for the WHO-PCDD/F (polychlorinated dibenzo-p-dioxins/furans)/TEQ (toxic equivalent) (Figure 5). Also, deviations for individual congeners fell within acceptable tolerances.
Figure 5 – Comparison of APGC-MS/MS results with PT assigned values and GC-HRMS mean values.The fish oil sample was analyzed after applying normal cleanup (A), cleanup without a carbon column (B), and cleanup without a carbon and florisil column (C). For fish oil sample C, considerable differences between the assigned value and the APGC-MS/MS result could be observed due to unresolved interferences on PCDFs. This indicated that cleanup is important for the quality of the analytical results (as in magnetic sector analysis), but carbon column cleanup may be removed.
Summary
Initial studies demonstrated sufficient sensitivity of the APGC-MS/MS for the monitoring of maximum and action levels for PCDD/F in feed and food matrices. As previously shown for other GC-MS/MS systems, the monitoring of two transition product ions provides selectivity for the analysis of PCDD/F, which is comparable to GC-HRMS at a resolution 10,000 (at 10% valley). The proposed amendments to EU regulations for the application of GC-MS/MS as a confirmatory method can also be met by the APGC-MS/MS system. The application of different cleanup methods for fish oil demonstrates that the extraction and cleanup steps have considerable influence on the quality of the analytical results.
Pesticides
Work carried out by Hernández et al.5 showed application of a pesticide method developed for the Xevo TQ-S APGC. The pesticides under study were sorted into three model groups as a function of their fragmentation pattern under the EI source:
Group 1—No M+ present
Group 2—Highly fragmented compounds
Group 3—EI spectra are similar.
Due to the nature of ionization by APGC, it is known that this technique can offer some significant advantages for difficult pesticide analysis by increasing the intensity of the molecular ion. Table 1 shows the relative intensity of the molecular (or quasi-molecular) ion in each different mode of operation.
Table 1 – Pesticides and relative concentration for each ionization technique*
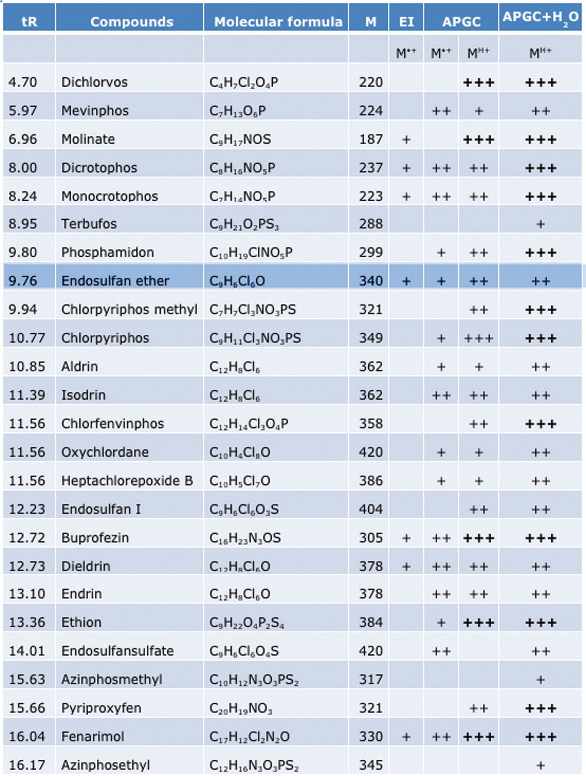
* +, very small peak; ++, clearly identifiable peak (>20%); +++, base peak
(or >80%).
It can be seen from the table that the majority of the compounds tested show a good intensity of the molecular (or quasi-molecular) ion. This is a significant advantage for the selection of the precursor ion for MS/MS analysis. When combined with the high sensitivity of the Xevo TQ-S and the ability to swap between LC and GC, it is a powerful system solution.
Preliminary experiments on linearity and precision of the APGC-MS/MS method have been performed with satisfactory results. Applicability of the method has been also tested in real samples comparing responses of fortified blank QuEChERS extracts and standards in solvent. No matrix-induced differences in ionization or fragmentation have been observed, and few, if any, interferences have been detected in MRM transitions (see Figure 6).
Figure 6 – Ethion in tomato extract and fenarimol in carrot extract with their respective solvent standards.Conclusion
The Waters Xevo TQ-S APGC offers significant advantages over traditional EI/GC/MS methods. Due to the nature of the ionization, reduced fragmentation is observed, and therefore a higher abundance of the molecular ion, leading to higher sensitivity and selectivity. The ability of the system to be rapidly changed from LC to GC makes it possible for the analyst to react to business demands with minimal down time. The Xevo TQ-S APGC offers highly sensitive, selective, easy-to-use, and flexible analytical performance.
References
- Munson, B. Chemical ionization mass spectrometry. Anal. Chem. 1971, 43(13), 28A– 43A; doi: 10.1021/ac60307a042, 1971.
- McEwen, C.; McKay, R. A combination atmospheric pressure LC/MS:GC/MS ion source: advantages of dual AP-LC/MS:GC/MS instrumentation. JASMS 2005, 16(11), 1730–8; doi: 10.1016/j.jasms.2005.07.005, 2005.
- Stevens, D.; Hsu, C.S. Atmospheric Pressure Gas Chromatography Mass Spectrometry (APGC/MS) for Novel Applications in Petroleum and Chemical Research. Oral presentation, 242nd American Chemical Society National Meeting, Denver, CO, 2011.
- http://eur-lex.europa.eu/LexUriServ/Lex- UriServ.do?uri=OJ:L:2012:084:0001:0022:E N:PDF.
- Hernández, F.; Beltrán Arandes, J. et al. Improved gas chromatography–tandem mass spectrometry determination of pesticide residues making use of atmospheric pressure chemical ionization. J. Chromatogr. A 2012, 1260, 183–92; doi: 10.1016/j. chroma.2012.08.009.
Mr. Jody Dunstan, BSc., MSc., CChem, MRSC, is Product Manager, Waters Corp., 34 Maple St., Milford, MA 01757, U.S.A.; tel.: +44 161 946 2472; fax: +44 161 946 2480; e-mail: [email protected]; www.waters.com.