Standard gel documentation has been performed for decades with some variation of the “camera on a stick” approach (see Figure 1), that is, the camera and lens are positioned above the target (Figure 1a) pointed downward at the sample, which is placed on either a UV transilluminator (i.e., ethidium bromide gels) or on a white light box (Figure 1b) for Coomassie- or silver-stained gels. Traditionally, the camera on a stick was set up in a darkroom with film processors and other photographic equipment. The darkroom provided a controlled environment that facilitated film processing postimage capture, and limited harmful exposure to other laboratory members when UV illumination was used.
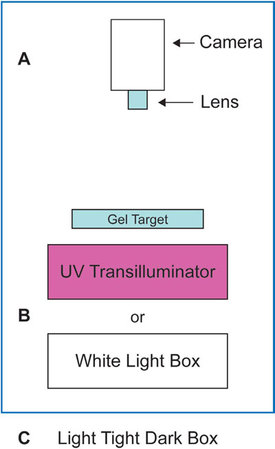
Figure 1 - Typical configuration for gel documentation.
More recently, with the advent of digital photography, most of the needs for a photographic darkroom have been eliminated. This, coupled with the typical space constraints of a research environment, have prompted the replacement of most photographic darkrooms with standalone imaging equipment that is contained within a “dark box” (Figure 1c) within which most gel documentation imaging applications can be performed. With this background, this article will examine some of the considerations one would look for when setting specifications for a gel documentation system for a laboratory.
Reliable image acquisition
Camera attributes
At the heart of any imaging solution is the camera. While high-end cooled charge-coupled device (CCD) cameras are required for imaging traditional colorimetric (i.e., Coomassie-stained proteins) or fluorescent (i.e., ethidium bromide-stained nucleic acids) gel labels, bit depth level is important, particularly when quantitative analysis is to be performed. The bit depth level refers to the number of discernible gray-scale levels that can be used to record the photonic illumination of any given pixel. While 8-bit to 14-bit systems provide the ability to take images of reasonable quality, 16-bit cameras are available, and are the best analytical solutions in this price range. Another concern when considering bit depth is the linearity. Typically, most 16-bit sensors can deliver 3–4 orders of linear dynamic range, which is optimal for accurate analysis of gel data.
The next important consideration is the number of pixels in the sensor. The recent pixel war driving traditional consumer camera markets (i.e., more pixels equal better resolution) has proven to be alive and well in scientific imaging. As such, despite the fact that a 1-megapixel (MP) sensor delivers more than enough resolution for the analysis of typical lane/band-type image data, recent entries into the scientific gel documentation market boast 7–10 MP cameras. Unfortunately, as the number of pixels that are fit onto fixed-sized silicon wafers is increased, the effective bit depth level goes down, creating a negative overall impact on the system. In general, cameras with 3–5 MP range, such as the Super CCD (Fujifilm Medical Systems USA, Woodbridge, CT), are more than adequate for standard gel documentation applications.
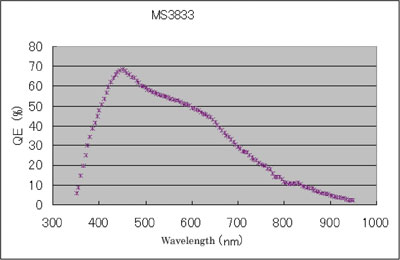
Figure 2 - Representative quantum efficiency curve for the MS3833 Super CCD.
The last aspect of CCD performance to consider from a gel documentation camera viewpoint is quantum efficiency (QE). The QE defines the percentage of photons of a particular wavelength hitting the photoreactive surface (i.e., CCD) that will produce an electron–hole pair. A representative QE curve for the Super CCD is presented in Figure 2. For most scientific-grade CCDs, sensitivity begins in the UV and extends into the near IR, making them useful for imaging most of the common colorimetric and fluorescent labels. Additionally, peak QEs in the range of 450–550 nm will provide good detection sensitivity for luminescent labels as well.
System attributes
Once a decision has been made regarding required camera specification, the next most important system component is the camera lens. The two main options are a variable zoom lens and a fixed focus lens. While variable zoom may seem attractive, it is usually configured on systems that have long fixed focal lengths. This results in longer pathways for photon scattering to occur, reducing sensitivity and resulting in increased exposure times, particularly when performing luminescence imaging. In contrast, the widest aperture (i.e., smallest f-stop) fixed focus lenses, such as the Fujinon F0.85 lens (Fujifilm Medical Systems USA), provides the greatest sensitivity and can be prefocused at staged focal lengths to improve work flow.
Beyond the lens, the darkbox should afford the user as many illumination configurations as possible. White and dia-illumination UV light sources for transillumination of gels provide the basic needs for traditional gel labeling protocols. These types of illuminators generally use standard fluorescent tube lights, which provide more than enough power for even the most demanding applications. On the other side, “epi” (i.e., illumination from above) can provide an enhanced array of excitation options for fluorescence labels in gels. The two main types of epi-illumination for excitation are provided by either filtering broadband light sources such as halogen or xenon, or by banking more refined output of light-emitting diodes (LEDs), which have the major advantage of generating consistent output. In effect, LEDs are either on or off, and if they are on, then the output will not vary by more than a few percent over the system’s lifetime. In contrast, broadband light sources can “burn” hot and cause deterioration of excitation filters, generating a much more variable output. No matter what form of illumination is chosen, the image field will not be uniform and will require correction in order to normalize signal intensity across the field of view.
Calibration
The first step in generating consistent digital image data for gel documentation is correction for the various sources of noise that arise in the sensor during the process of accumulating and recording photons. These include photon shot noise, sensor read noise, and thermal noise, as well as noise created by pixels that are either locked on “hot” or off “cold” inappropriately. These types of calibrations are usually performed by capturing dark shot images (i.e., images collected with the shutter closed) at specific time intervals and/or bin states, and either subtracting or dividing these normalizing images from subsequent images captured under identical conditions.
The final calibration relevant for gel documentation systems addresses all component variation distal to the shutter, including the lens prescription, variation in the optical path for the field of view, and variation in any illuminating light sources. All of these factors can create tremendous variability and need to be consistently corrected by the generation of reliable image data and application of appropriate algorithms.
Consistent image acquisition
Operational qualification
Performing general gel documentation using GMP/GLP practices helps ensure that reliable results can be consistently generated. Toward that end, a system should be able to determine that the necessary calibration files for reliable system performance have been recorded and that they are accessible should a specific application be invoked. These self-checks should be performed automatically upon system startup, and should be available for documentation purposes as either a printed report or saved in electronic format. This provides a means of verifying that no changes in system function, per a given application, were noted on a specific date, providing greater confidence that any changes in image data are a result of experimental design or production variation. This is particularly important in QA laboratories, where results could determine whether a product is released for therapeutic applications.
Software programming
Once all calibrations and system operation are reliably set up and documented, the ability to acquire images consistently will be a function of user interface with the acquisition software. The key here is automation, and in this regard the ability to template sets of acquisition parameters (i.e., exposure time, camera f-stop, light source) into a single user-selectable parameter should be carefully considered. While these types of system attributes come at a cost, they do provide a means of ensuring that technicians or other operators who do not understand the theory behind the system are not required to make acquisition decisions on their own that can have significant impact on the recorded image dataset.
Controlled access and audit file history
21 CFR Part 11
The need for tighter controls around the acquisition and handling of digital image data is being accelerated in part by the FDA’s Title 21 CFR Part 11 of the Code of Federal Regulations, which deals with electronic records signatures. Part 11, as it is commonly called, defines the criteria under which electronic records and electronic signatures are considered to be trustworthy, reliable, and equivalent to paper records. In practice, setting up a laboratory to function in this type of GMP/GLP environment makes sense even for basic research.
The key components necessary for operating within a Part 11 environment are controlled access and traceability. On the access side, both data acquisition and analytical analysis software should provide a means of administrative oversight. The system administrator should be able to set levels of user interaction such that passwords are required to gain access to the software (and ultimately the system), and unauthorized users may not. On the traceability side, software should provide a means for tracking changes and auditing image data to ensure that any modifications made either to the image or subsequent analysis can be determined. Again, this type of functionality comes at a cost; thus systems that are flexible in adding these features as modular components will provide users with the most options moving forward.
Summary
Gel documentation has traditionally been thought of as a low-technology laboratory application. However, the need for reliable and consistent image acquisition as well as documentation of system performance and subsequent analytical analysis require that a suitable software interface be in place to meet these demands. Since there is a cost associated with this functionality, systems that are modular and that can be upgraded empower users to accessorize their systems with the most cost-effective options to meet the needs of the laboratory.
Dr . Pizzonia is Director, Applications, FUJIFILM Medical Systems USA, Inc., Life Sciences Group, 11 Research Dr., Ste. #3, Woodbridge, CT 06525, U.S.A.; tel.: 800-446-5450, ext. 3460; 203-276-3460; fax: 203-363-3869; e-mail: [email protected].