Polymeric materials, in a general sense, are viscoelastic in nature since they exhibit a strong dependence of deformation and flow on time and temperature. Performance of these materials can only be evaluated by testing the materials under conditions that the material would encounter in real-time applications. This poses serious problems in evaluating materials that would need to be in service over very long periods of time, or if the material undergoes deformations (rates) over a wide range. For example, it would be impossible to evaluate the flow behavior of materials in solar panels over the span of time they are designed for (20–30 years). Similarly, aircraft/spacecraft materials that are rated for service up to thousands of hours can be evaluated. Measurements taken using an instrument at a set temperature usually cover a range of three to four orders of magnitude of time or frequency. This range is usually not sufficient to evaluate material viscoelastic behavior in the complete range of frequencies required, from the low-frequency terminal zone to the high-frequency plateau zone.
Time–temperature superposition
Time–temperature superposition (TTS) has been used over the last few decades to solve the kind of problems mentioned above. This technique is well grounded in theory and applies to a wide variety of hydrocarbon materials that are thermorheologically simple. Thermorheologically simple materials are those in which all relevant relaxation and retardation mechanisms as well as stress magnitudes at all times and frequencies have the same temperature dependence. The theory and mechanisms that form the basis for the TTS technique have been well documented in the literature.
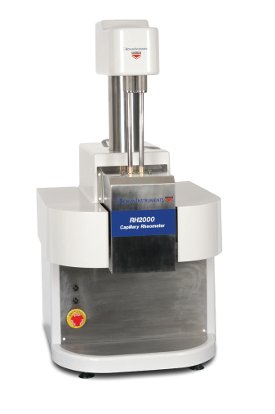
Figure 1 – RH2000 Dual Bore Capillary Rheometer.
The application of TTS to predict the creep behavior of materials over a long period of time has been in practice for a few decades. However, predicting material flow behavior and viscosity values of materials at extremely high deformation rates is a relatively uncharted territory. This study demonstrates the use of capillary rheology using an RH2000 Dual Bore Capillary Rheometer (ATS RheoSystems, Bordentown, NJ) (Figure 1) equipped with specially fabricated dies providing length/diameter (L/D) ratios >400 to measure viscosity profiles of motor oils up to high shear rates of 1,000,000 s–1. The data sets at lower temperatures (100, 110, 120, and 130 °C) are superposed onto the data set at 150 °C using the TTS technique (developed by Dr. Henning Winter at IRIS Development Inc. [Amherst, MA]) in order to predict the true viscosity behavior of the oil at high shear rates (up to ~1 million s–1) at 150 °C. This study demonstrates that the TTS technique can be used to predict such data with reasonable accuracy.
Measurement of rheological properties using a capillary rheometer
The RH2000 Capillary Rheometer was used to measure the rheological properties of samples over a wide range of shear rates and test conditions. The rheometer can measure shear rates from 0.01 to over 10,000,000 s–1. A wide variety of die sizes and L/Ds are available. Corrosion-resistant sample chambers, pistons, and dies are also offered. Temperature control is better than ±0.1 °C. Other options include: 1) automatic cleaning, 2) nitrogen blanket, 3) pressure-volume-temperature system, 4) thermal conductivity system, 5) slit die geometry, 6) die swell, 7) stretching unit, and 8) extensional viscosity by the Cogswell method. The FlowMaster software (Malvern Instruments Ltd., Westborough, MA) can be run in manual, plateau, and automatic equilibrium stress prediction (ESP) mode. The ESP mode allows experiments to be run without user intervention. Other available features in the software are the Weissenberg-Rabinowitsch and Bagley corrections, advanced curve-fitting module, elongational viscosity module, wall slip module, and thermal degradation analysis module. Data exportation to other Windows programs is included as standard.
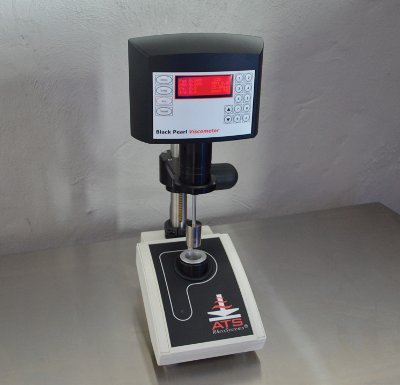
Figure 2 – BLACK PEARL Viscometer.
One of the limitations of capillary rheometers in general is that very low pressures are developed at low shear rates when testing low-viscosity fluids. The low pressures sometimes tend to fall close to the limitations of the capillary rheometer’s pressure transducers, rendering data with low signal-to-noise ratios. Therefore, data at such low shear rates need to be verified using other viscometers/ rheometers. The low shear data in this study were verified using a BLACK PEARL Viscometer (ATS RheoSystems) (Figure 2), which can be used to accurately measure the flow profiles of low-viscosity fluids at lower shear rates (Figure 3). The low-shear-rate viscosity profile plateau of commercial multiweight motor oil using the BLACK PEARL Viscometer is shown in Figure 3.
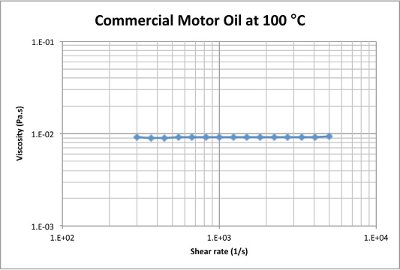
Figure 3 – Low-shear-rate viscosity profile plateau of commercial motor oil at 100 °C measured on the BLACK PEARL Viscometer.
The BLACK PEARL Viscometer can also be used to study the mixing, stirring, and pumping behaviors of coatings, emulsions, and dispersions. The design incorporates a built-in Peltier temperature control system that permits isothermal, step, and/or ramp temperature profiles in the range –10 to 120 °C. The DIN standard measuring systems of cone and plate, parallel plate, and bob and cup, coupled with a wide shear rate (angular velocity 0.01–200 rad/ sec), torque (0.005–20 mNm), and temperature range provide a measurable viscosity range from 0.5 to 1E08 cP. Additional measuring systems allow for a wider range of product investigation and experimentation. These include bladed “vane” tools and narrow gap couette systems. The narrow gap couette system utilizes a smaller annular gap (1.25 mm for the DIN standard vs 0.5 mm for the narrow gap system) to optimize testing of low-viscosity samples.
Experimental: Steady shear rate rheological measurements
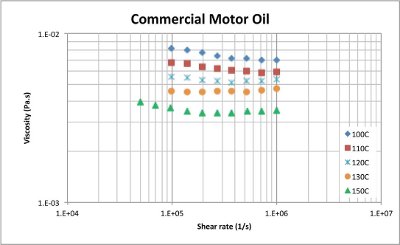
Figure 4 – Steady-state shear viscosity profiles of the commercial multiweight motor oil at 100, 110, 120, 130, and 150 °C run with the RH 2000 Capillary Rheometer.
Steady shear rate rheological measurements of commercially available multiweight motor oil, using an RH2000 Capillary Rheometer with specially fabricated die of dimensions 0.147 × 65 mm (L/D = 400), are presented in this study. A 69-MPa pressure transducer was used for all experiments. The shear rates used in this study ranged from ~50,000 to 1,000,000 s–1. Viscosity profiles of the oil at 100, 110, 120, 130, and 150 °C are presented. Viscosity profiles at low shear rates were verified with the BLACK PEARL Viscometer using a narrow gap cup and bob measuring system with a 25-mm-diameter bob and a 26-mm-diameter cup.
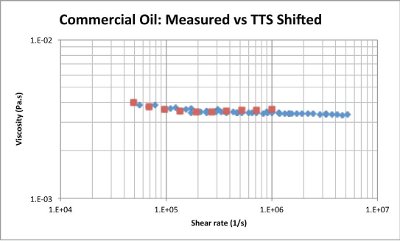
Figure 5 – Predicted viscosity data of the commercial oil over a large range of shear rates at 150 °C using TTS compared to original viscosity profile using capillary rheology.
Time–temperature superposition was used to estimate viscosity values of the oil at extremely high shear rates using a commercially available TTS data shifting software package (IRIS). TTS shifting gives viscosity data at higher shear rates with reasonable accuracy while eliminating the upturn in viscosity at high shear rates caused by turbulence. Viscosity profiles of respective oils at 100, 110, 120, and 130 °C were shifted to superpose onto the viscosity profile at 150 °C to generate the master curve. The master curve predicts the viscosity profile of the oil up to high shear rates (1,000,000 s–1).
Results and discussion
The commercial multiweight oil viscosity profiles at 100, 110, 120, 130, and 150 °C are presented in Figure 4. The oil exhibits shear thinning behavior in the temperature range measured. The high end shear rates measured experimentally are unique to this study. Viscosity measurements up to the high shear rates represented in this study are achievable by using a die with high L/D ratio (>400) with a novel die design. The die fits into a specially designed die holder, which can be modified to fit any commercially available capillary rheometer. Normally, commercially available capillary rheometers are not equipped to measure viscosities of low-viscosity fluids at high shear rates.
As expected, the oil viscosity decreased with the increase in temperature. It should be noted that the viscosity profiles at 120 °C and above showed an upturn at shear rates above approximately 500,000 s–1. This upturn is due to the onset of secondary flows (turbulence). Turbulent flow manifests itself as an upturn in viscosity due to additional resistance to flow. The use of the high L/D ratio dies pushes the onset of turbulence to shear rates of ~500,000 s–1, which otherwise would set in at much lower shear rates leading to misleading viscosity values. This in turn would make it impossible to estimate viscosity values at high shear rates using TTS.
Figure 5 presents the master curves obtained by superposing the viscosity profiles of the commercial multiweight non-Newtonian oil (from Figure 4) at 100–130 °C onto that at 150 °C. As expected, the non-Newtonian oil exhibits slight shear thinning behavior, as would be expected from the experimental data. Figure 5 also compares the experimental data set measured at 150 °C with that of the master curve generated using TTS. It is demonstrated that the upturn in viscosity due to secondary flows was eliminated, and viscosity values of the oil up to 5,000,000 s–1 were predicted accurately using TTS.
Conclusion
This study emphasizes the importance and explains the applications of high-shear-rate viscosity measurements. A novel technique to measure low-viscosity fluids at high shear rates (~50,000–1, 1000,000 s–1) was developed using capillary rheology. The difficulty in achieving reliable viscosity measurements at high shear rates (300,000–1,000,000 s–1) was addressed, and a method to estimate the viscosity behavior of low-viscosity fluids with reasonable accuracy up to shear rates of 5,000,000 s–1 was established (time–temperature superposition). It has been demonstrated that the time–temperature superposition technique, along with the modified high L/D die assembly, alleviates the problem of errant viscosity numbers at high shear rates (>300,000 s–1) due to the onset of turbulent flow. This study is expected to have a prolific impact on low-viscosity high-shear-rate applications such as oil flowing through engine parts, paint spray guns, and petroleum pipelines, among other applications in various industries.
Jeshwanth K. Rameshwaram, Ph.D., and Tien T. Dao, Ph.D., are with ATS RheoSystems, 231 Crosswicks Rd., Bordentown, NJ 08505, U.S.A.; tel.: 609-298-2522; e-mail: www.atsrheosystems.com; [email protected]. The authors sincerely appreciate all the cooperation extended to them by the developer of the IRIS software, Dr. Henning Winter at IRIS Development Inc. (Amherst, MA), regarding applying the time–temperature superposition to extend the shear rate range in this study using the IRIS software.