Most organic molecules, especially biomolecules, absorb light in the UV range with the response being proportional to their concentration. This has led to UV spectrophotometry becoming widely adopted as a label-free method of determining the concentration of proteins and nucleic acids in various applications including enzyme assays and cell counting. In analytical biochemistry, UV absorption instruments are typically incorporated as flow-through UV detectors that monitor and quantify molecular species after they have been separated using either liquid chromatography or capillary electrophoresis (CE) techniques.
An important advance in UV detector development was made in the 1980s, with photodiode arrays (PDAs) replacing photomultipliers in spectrophotometers. In conjunction with a diffraction grating, these linear arrays enable continuous signal monitoring over a broad wavelength range.
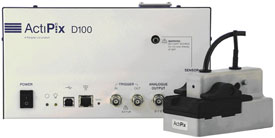
Figure 1 - ActiPix D100 detector.
In 2007, the world’s first UV area detector, the ActiPix D100 (Figure 1) (Paraytec Limited, York, U.K.) was developed, which uses an active pixel sensor (APS) to image an area rather than the line characteristic of PDA detectors. One of the key advantages of using an APS detector is that signal referencing can be carried out using pixels in the second dimension such that any light source fluctuations have minimal impact on baseline stability. This internal referencing results in high performance when incorporated with flow-through devices such as LC and CE.1,2
Rather than a diffraction grating, the system employs easily changeable wavelength filters; thus the sample band can be imaged continuously along the entire length of the 9-mm sensor. Capillaries are used as both sample cells and optical elements that provide inherent spatial separation of the sample and reference beams. Additionally, multiple capillaries can be imaged simultaneously, enabling multiple reactions or separations to be monitored at the same time.
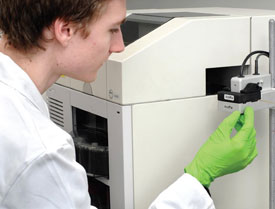
Figure 2 - External positioning of ActiPix to a commercial CE system.
The ActiPix offers flexibility and adaptability, with available cartridges designed for different applications (Figure 2). It can be used as an on-line detector with any capillary system, e.g., CE, nanoLC, or microflow injection analysis (μFIA), or can be coupled with micrototal analysis systems (µTAS) and lab-on-a-chip devices. Switching from one mode to another is very fast, and is achieved by simply interchanging cartridges designed for different applications. The pixel maps created by the signal and referencing regions are automatically identified each time a new capillary arrangement is positioned in the imaging area, without the need for user intervention.
While lab-on-a-chip devices have received much media attention, lab-on-capillary setups have largely gone unnoticed. This is particularly surprising since, unlike the channels in chips, capillaries have well-defined cross-sections over the long distances required to provide high-resolution separations of complex mixtures. Furthermore, lab-on-a-chip devices can be difficult to manufacture and are generally not amenable to direct detection of products and reactants using UV.
The ActiPix D100 has already been used in diffusion measurements, proteomics experiments, monitoring of in-capillary reactions, and kinetic measurements. It is especially important for a creative analytical scientist that the detector be able to provide the flexibility to exploit integration with other instruments and to implement nonstandard arrangements. For example, the way in-capillary biochemical assays can be multiplexed by imaging species separation in parallel capillaries using a standard CE instrument has been demonstrated.2,3 Such an arrangement is easily achieved using off-the-shelf connectors, without the need for any special fabrication. Use of a looped capillary arrangement in a CE experiment permits multiple window sections of a single capillary to be viewed in the imaging area,4 enabling the velocities of species originating from any point to be readily measured.5 This feature is very useful for monitoring in-capillary biocatalytic processes.
Multicomponent on-capillary biocatalytic assays
The ActiPix detector can take snapshots at multiple points in a looped lab-on-capillary flow line to allow biochemical assays on nanoliter sample volumes. The first example demonstrates the feasibility of assessing the substrate specificity of an enzyme using a multicomponent biocatalytic assay. The process involves monitoring multiple detection windows on a single looped capillary that enables the separated products of an enzyme-catalyzed reaction to be observed.
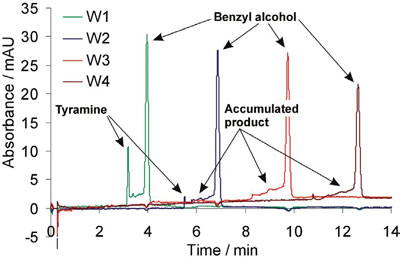
Figure 3 - Continuous in-capillary assay of tyramine oxidase. The enzyme was dissolved in the BGE composed of 25 mM phosphate buffer at pH ~7, while the sample containing tyramine and benzyl alcohol was injected as a plug. During the run, both voltage (+25 kV) and pressure (+50 mbar) were applied. The capillary contents are visualized at four sections on the capillary (W1, W2, W3, and W4, located 40, 70, 100, and 130 cm from the capillary inlet, respectively; total capillary length 150 cm, i.d. 75 μm, o.d. 193 μm). Detection wavelength: 200 nm.
A 150-cm-long capillary was initially filled with background electrolyte (BGE) containing the enzyme, tyramine oxidase. Next, a mixture of tyramine and benzyl alcohol was injected. Upon application of a small hydrodynamic pressure and voltage, the compounds started to separate with tyramine oxidation taking place at the same time. This is shown by a decrease in both amplitude and area of the peak of tyramine as it traverses four detection windows (Figure 3), while the peak area of the flow front marker, benzyl alcohol, is unaffected.
This success was built upon with the development of a method to assess the specificity of an enzyme against a range of putative substrates.4 The method also uses four detection windows placed side by side across the imaging area. This time, a complex mixture of substrates was injected into the capillary prefilled with a BGE containing the enzyme. The progress of the various reactions was subsequently monitored as the putative substrates flowed past each of the capillary windows.
The method requires only minimal amounts of reagent, making it well suited for evaluating enzyme specificity if only small amounts of the enzymes are available. Since up to eight capillary windows can be imaged with the ActiPix D100, it is possible to carry out runs in a multiplexed fashion using two looped capillaries with four windows placed side by side across the imaging area.
Protein sizing
The second application demonstrates a simple protein sizing protocol. Unlike dynamic light scattering (DLS), the sizing method is applicable to both small and large molecules and does not suffer from the inherent bias toward large molecules that hampers the use of light scattering techniques. The method relies on measuring the peak broadening that occurs due to Taylor dispersion6,7 as a sample plug travels along a capillary. Since the peak area can also be used to quantify protein concentration, the method is suitable for monitoring the progress of recombinant protein production processes.
When a liquid flows through a capillary or tube, a velocity profile develops, with the liquid flowing faster in some radial positions than others. This leads to particles in the liquid being dispersed along the tube axis, with larger particles being affected more than smaller ones. By measuring the change in width of a UV peak as a solution flows past a number of capillary windows, particle sizes can be calculated.
In a typical experiment, a commercial CE system is used to inject the sample and to apply hydrodynamic pressure on a protein plug. The sample is pushed through the capillary loop and the width of the sample band recorded at two detection windows before and after the loop.1 The increase in peak standard deviation is inversely proportional to the square root of the diffusion coefficient, and because the diffusion coefficient is inversely proportional to the hydrodynamic radius, the approach provides a simple way to measure the size of molecules using nanoliter amounts of sample.
Using this technique, the hydrodynamic radius, Rh, of bovine serum albumin (BSA) at a pH of 8.92 was determined to be 3.22 ± 0.14 nm. The conformation, and therefore size, of BSA has been shown to be pH dependent. Nevertheless, this figure agrees well with protein crystallography data that suggest the hydrodynamic radius to be 3.7 nm in the pH range 4–8.8
Another advantage of using a CE instrument with the ActiPix for protein sizing is that the analytes in a mixture can be separated in the capillary prior to sizing in the loop. Alternatively, proteins can be separated by capillary LC before size analysis.
In the case of solutions of purified single components, the analysis can be carried out using an even simpler setup. The ActiPix capillary loop sizing system can be connected to any pump providing constant low-volume flow rate, for example, a syringe pump, an LC pump, or any miniature pump designed for use with microfluidics. In the most economical variant of the sizing setup, gravity-driven siphoning can be used to move the sample zone along the capillary. In all cases, quantitative information on hydrodynamic radii can be gained within minutes and using nanoliters of samples of nonlabeled UV-VIS-absorbing analytes.
The use of the technique is currently being extended by a multidisciplinary U.K. R&D consortium led by Paraytec with partners Avecia Biologics Ltd. (Billingham), Intertek ASG (Manchester), Eli Lilly and Company Ltd. (Liverpool), Lonza Biologics Plc (Slough), and the University of Bradford (Bradford). The project, entitled “Novel Analytical Instrumentation to Detect Aggregation in Biopharmaceutical Processing,” is being funded by research grants from the U.K.’s Technology Strategy Board (TSB) and the Engineering and Physical Sciences Research Council (EPSRC).
Lab-on-capillary
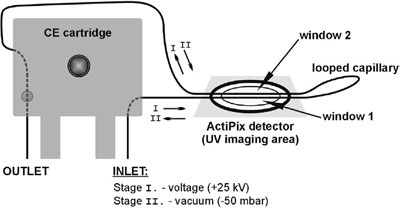
Figure 4 - Experimental setup for the simultaneous reaction, separation, and sizing.
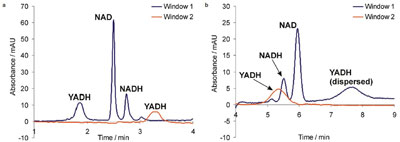
Figure 5 - Simultaneous reaction, separation, and sizing: a) first stage, plug–plug EMMA process; b) second stage, sizing of the enzyme (YADH). Detection wavelength: 200 nm. See text for further explanation.
The versatility of using ActiPix and a CE system is illustrated in the final example, which combines a sequence of three processes (enzymatic reaction, separation, and protein sizing) all taking place in a single looped capillary format (see Figure 4).
Figure 5a shows the signals registered during the first stage of the protocol, plug–plug electrophoretically mediated microanalysis (EMMA) of yeast alcohol dehydrogenase (YADH), including reaction and separation of the substrate and product cofactors and the enzyme.3 Figure 5b shows the signals used for sizing of YADH according to the same principles as those applied to the sizing of BSA (see above).
At the beginning of the experiment, a sandwich injection was carried out in the sequence: nicotinamide adenine dinucleotide (NAD), BGE, YADH (50 mbar, 5 sec each). The voltage (+25 kV) was then switched on to initiate zone overlap and the in-capillary synthesis of nicotinamide adenine dinucleotide, reduced form (NADH). This was followed by separation of YADH, residue NAD, and the product NADH. All three species traversed the first detection window of the loop (window 1); YADH (the fastest migrating species) also traversed window 2 (Figure 5a).
After 4 min, the voltage was switched off, and reduced pressure (–50 mbar) was applied to the inlet vial in order to induce hydrodynamic flow in the opposite direction, i.e., from the capillary outlet to the inlet. The YADH zone now traversed window 2 first, and then window 1, with evident band broadening of the peaks as they passed the windows (Figure 5b). The biocatalytic conversion can be calculated from the data obtained in the first stage of the experiment (Figure 5a), and the hydrodynamic radius of YADH calculated from the second stage (Figure 5b).
The protocol can be developed further to avoid changing the direction of the BGE flow in the capillary by using three or four loops in the setup. If the enzyme is impure, the protocol can be optimized so that the impurities are separated from the main component in the separation stage (with voltage) to avoid interference during the sizing stage (with vacuum).
Conclusion
The ActiPix is not only applicable as a detector to be used in combination with capillary separations, but its 2-D visualization capabilities can also be used as a quantitative UV imaging system. This is a real benefit in comparison with single-point UV detectors, and therefore the system will find many exciting applications in bioscience.
Assays similar to those described in this article will assist with the characterization of promiscuous enzymes destined for applications in biocatalytic synthesis and help identify those compounds unaffected by enzyme-dependent mechanisms of microbial drug resistance. The multisection detection also offers the possibility to control migration of peaks over a long capillary, a factor that is especially important when studying molecular interactions during affinity capillary electrophoresis (ACE) experiments.
The lab-on-capillary approach described in this article shows how structural and reactivity information about an enzyme may be gathered during a single experiment. The concentration of the enzyme present can be accurately calculated from the peak area, the activity of the enzyme can be measured by substrate conversion, and this activity can be linked to the structural information gained by the calculation of the hydrodynamic radius.
As protein structures can change in the presence of substrates and with varying pH,8 the ability to collect enzyme activity and structural data during the same experiment is crucial to furthering our knowledge about biological processes. Since the method is nondestructive, the analytes and enzyme can be recovered at the end of the experiment if necessary.
The sizing protocol may also be suitable for the sizing of nanoparticles, including quantum dots and polymers. This application of the ActiPix detector could serve as an enabling technology for the emerging field of nanotoxicology, since Choi et al.9 have suggested that the hydrodynamic radius of a nanoparticle is a key factor in the kidney’s ability to remove it from the body.
References
- Goodall, D.M.; Urban, P.L. Int. Labmate 2007.
- Urban, P.L.; Goodall, D.M.; Bergström, E.T.; Bruce, N.C. Electrophoresis 2007, 28, 1926–36.
- Urban, P.L.; Goodall, D.M.; Bergström, E.T.; Bruce, N.C. J. Chromatogr. A 2007, 1162, 132–40.
- Urban, P.L.; Goodall, D.M.; Carvalho, A.Z.; Bergström, E.T.; Van Schepdael, A.; Bruce, N.C. J. Chromatogr. A 2008, 1206, 52–63.
- Urban, P.L.; Bergström, E.T.; Goodall, D.M.; Narayanaswamy, S.; Bruce, N.C. Analyst2007, 132, 979–82.
- Bello, M.S.; Rezzonico, R.L.; Righetti, P.G. Science 1994, 266, 773–6.
- Cottet, H.; Biron, J.P.; Martin, M. Anal. Chem. 2007, 79(23), 9066–73.
- Brownsey, G.J.; Noel, T.R.; Parker, R.; Ring, S.G. Biophys. J. 2003, 58, 3943–50.
- Choi, H.S.; Liu, W.; Misra, P.; Tanaka, E.; Zimmer, J.P.; Ipe, B.I.; Bawendi, M.G.; Frangioni, J.V. Nat. Biotechnol. 2007, 25, 1165–70.
Prof. Goodall is CSO, Paraytec Limited, 1a St. George’s Place, Tadcaster Rd., York YO24 1GN, U.K.; tel.: +44 1904 526275; fax: +44 1904 652101; e-mail: [email protected]. Dr. Urban is PDRA, Faculty of Biology, Warsaw University, Warsaw, Poland. Dr. Urban would like to thank the Foundation for Polish Science (FNP) for the stipend “START” received in 2008.