With the 2012 Olympics fast approaching, many still remember the trouble caused by one of the most notorious recombinant proteins, human growth hormone (HGH). Once used as an illegal anabolic agent to boost athletes’ performance, today the therapeutic uses of HGH in the field of medicine are extensive. In fact, recombinant proteins such as human hormones, enzymes, and cytokines are the focus of many research projects across the globe and are responsible for producing a wide range of medicines. Recombinant proteins are manufactured from DNA introduced into a vector system, such as bacterial plasmids from the bacterium Escherichia coli. Although the E. coli system possesses many advantages for producing recombinant proteins, there are a range of limitations associated with its use, ranging from low protein expression to lack of post-translational protein modification. This article describes a method for the optimization of soluble protein expression in E. coli using the MaxQ® 8000 refrigerated stackable shakers (Thermo Fisher Scientific, Asheville, NC). When combined with good experimental design, the protocols show that protein expression can be improved rapidly, with differences observed over a period of hours rather than days.
Role of the plasmid
E. coli is in many respects an ideal system for the production of recombinant proteins. Found ubiquitously in nature, with most strains harmless and part of the normal flora of the gut,1,2 the bacterium possesses within its cell wall small bundles of DNA called plasmids. These DNA molecules are separate from, and can replicate independently of, the chromosomal DNA.3 As a result, plasmids provide a method for horizontal gene transfer within a population of microbes and can confer selective advantage under certain environmental conditions. A good example of this is resistance to naturally occurring antibiotics, which are carried by genes on the plasmid. Restriction enzymes are used as “molecular scissors” to cut the plasmid DNA and allow the recombinant DNA to be integrated into the plasmid’s double-stranded circle.
Although E. coli is commonly used for the production of recombinant proteins, there are a range of issues associated with its use, such as the length of DNA inserts that can be added to the plasmids. Prokaryotes such as E. coli also do not carry out the same level of post-translational modifications to the protein as human eukaryote cells (such as glycosylation and phosphorylation). In addition, protein products can become insoluble and form what are known as inclusion bodies—nuclear aggregates of proteins in the bacterial cytoplasm. However, the versatility and ease of use make this a choice vector system, capable of producing large quantities of soluble protein in a short amount of time, without the need for extensive equipment or skills. In order to maximize the potential of using E. coli as a vector system, a variety of options are available to increase protein expression in order to optimize experiments and enhance the final protein levels.
Low protein expression: Tackling the issue
Genetically engineered fusion tags can improve the variable yield and poor solubility of many recombinant proteins. Fusion tags can be broadly classified into two categories: first, affinity tags that aid in purification but do not enhance the solubility of the proteins substantially. These can be defined as exogenous amino acid (aa) sequences that bind with high affinity to a chemical ligand or an antibody. The second types of fusion tags are solubility-enhancing tags that specifically enhance the solubility and recovery of functional proteins. Solubility-enhancing tags are generally large peptides or proteins that increase the expression and solubility of fusion proteins.4 When designing a fusion tag, careful consideration must be given to how the tag will be removed to produce native proteins without any unwanted sequences attached.
Another issue that may affect protein expression is that the use of codons in E. coli DNA is different than that of the inserted human gene. There is a large difference in the codons most commonly used in eukaryotes compared to those preferred by the prokaryotic E. coli. This has been tackled by two approaches, either through the use of E. coli strains that encode tRNAs that are rare in the bacterium, but are used frequently in other organisms, or through the use of synthetic genes that have been codon-optimized via complex algorithms that take into account the codon bias of E. coli.
Testing for optimal conditions
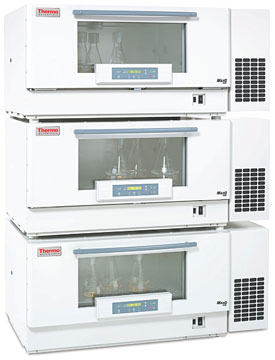
Figure 1 - Thermo Scientific MaxQ refrigerated stackable shakers.
Although the fusion tags and codon optimization techniques mentioned above can help in increasing the levels of recombinant protein expressed, there are also simpler and more cost-effective methods available. The different variables for a recombination experiment, such as the temperature of induction, length of induction, and type of media used in the experiment, can all have an effect on the amount of soluble recombinant protein obtained. In order to maximize yield of protein, the best conditions for each individual recombinant protein can therefore be determined; however, this can often be a time-consuming process. In previous studies, laboratory shakers have been shown to help improve protein yields.5 In order to ascertain which experimental conditions can improve protein expression, MaxQ 8000 refrigerated stackable shakers (Figure 1) were used. The shakers helped to reduce the time and effort needed to optimize the expression of a target protein by simultaneously testing eight expression conditions in a period of hours rather than days.
MaxQ 8000 refrigerated stackable shakers are well suited for high-volume research or scaleup work and provide three times the capacity of a floor shaker in virtually the same footprint. The units are built for sensitive eukaryotic culture applications and are equipped with contamination-reducing HEPA filtration and tight temperature uniformity. In the experiment, the target His-tagged recombinant protein used was a 10.5-kDa, multistranded β barrel with an intervening helix insert region (referred to as protein X). The protein was expressed under the control of a T7 promoter, and the stackable shakers were used to test growth temperatures, length of induction, and different media in just hours, rather than the many days normally needed to perform these studies. A Design of Experiments (DoE) process was integrated into the experiment to ensure that both the individual and combinatorial effects of each of the experimental factors could be analyzed statistically.
Table 1 - Experimental conditions: Three-factor, two-level experimental design
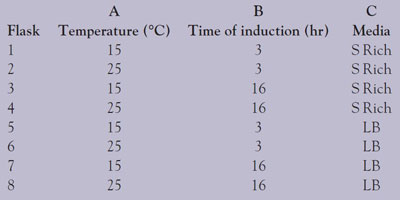
Eight flasks were seeded with 10 mL from a 16-hr, 100-mL starting culture. These 2-L flasks were grown at 30 °C and spun at 200 rpm until there were enough cells to start the protein production. At that point, isopropyl β-D-1-thiogalactopyranoside (IPTG) was added to trigger protein production, and the flasks were incubated under the conditions detailed in Table 1.
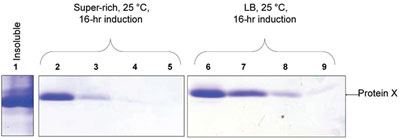
Figure 2 - SDS-PAGE gels that represent the findings of the study. The gels were scanned and the band densities evaluated. The insoluble fraction contains the majority of the expressed protein. Lanes: 1) Insoluble protein; 2, 3, 4, 5) sequential elutions with 250 mM imidazole for sample incubated in super-rich media at 25 ºC with a 16-hr induction (flask 4); 6, 7, 8, 9) sequential elutions with 250 mM imidazole for sample incubated in LB media at 25ºC with a 16-hr induction (flask 8).
The cells were harvested by centrifugation. The remaining cell pellets were then frozen at –80 °C to aid lysis. Cells were lysed in a bacterial protein extraction reagent with added enzymes, and the lysate was cleared by further centrifugation. Each lysate was then purified and protein X was washed and eluted from the chromatography columns. Fractions were analyzed by sodium dodecyl sulfate-polyacrylamide gel electrophoresis (SDS-PAGE) (see Figure 2) and the quantities of protein present were determined by densitometry. Measurements were performed in duplicate to assess error. The data were then analyzed statistically using the Design-Expert® 7 workstation (Stat-Ease, Inc., Minneapolis, MN).
Maximizing soluble protein expression
All three factors (temperature, time of induction, and media) were shown to have statistically important effects on the levels of soluble protein X expression. The screening identified conditions of expression that resulted in a greater than twofold improvement in the yield of protein X; however, the Super Rich medium had an overall lower expression yield. For this medium, temperature and time of induction both had a positive effect on the soluble expression of the protein, but the effect was not cumulative. In fact, when both temperature and time of induction were increased, the solubility of the protein dropped. For the LB medium Luria-Bertani, however, increased temperature and time of induction had an additive effect, increasing the levels of soluble protein expression.
In summary, the MaxQ 8000 refrigerated stackable shakers proved to be an effective instrument for determining which experimental variables had an effect on the levels of soluble protein expressed by the E. coli vector system. Valuable time was saved since only a few hours, rather than several days, were required to determine the best conditions for recombinant protein expression.
References
- Bentley, R.; Meganathan, R. Biosynthesis of vitamin K (menaquinone) in bacteria. Microbiol. Rev. 1982, 46(3), 241–80.
- Hudault, S.; Guignot, J. et al. Escherichia coli strains colonising the gastrointestinal tract protect germfree mice against Salmonella typhimurium infection. Gut 2001, 49(1), 47–55.
- Lipps, G., Ed. Plasmids: Current Research and Future Trends. Caister Academic Press, Norfolk, U.K., 2008.
- Chelur, D.; Unal, O. et al. Fusion Tags for Protein Expression and Purification [Online] 2008, Available: http://biopharminternational.findpharma.com/biopharm/Upstream+Processing/Fusion-Tags-for-Protein-Expression-and-Purification/ArticleStandard/article/detail/522166; BioPharm International.com [10 January 2011].
- Peti, W.; Page, R. Strategies to maximize heterologous protein expression in Escherichia coli with minimal cost. Prot. Expres. Purif. 2007, 51(3), 1–10.
Dr. Schofield is Research Scientist II, Thermo Fisher Scientific, 3747 Meridian Rd., Rockford, IL 61105-0117, U.S.A.; tel.: 815-968-0747; e-mail: [email protected].