Historically, the scientific method had been based upon the reduction of complex systems to their simplest forms in order to understand their individual processes. In biology, a whole animal will be reduced to its constitutive organs, these organs to individual tissues, and then individual areas to single cell types. The isolation of a single cell type and the use of artificially created immortalized cell lines or cancer cell lines are the foundation of modern cell culture. Although the first attempts at culturing cells in vitro took place in the early 1900s on plasma clots, cell culture as we know it today did not really exist until the 1950s, with the introduction of the laminar flow hood, fetal bovine serum, and sterilizing filters. Sixty years of culturing individual cell types on glass or plastic surfaces using fetal bovine serum have shown it to be a powerful technique for studying their functions. However, cells do not grow on plastic in nature, and certainly cell-matrix and cell-to-cell interactions play a key role in generating the 3-D biological organization we call multicellular “life.”
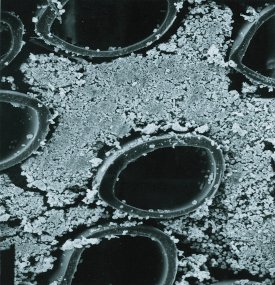
Figure 1 - Cross-section of a hollow fiber bioreactor showing the fibers and lymphocytes in suspension growing around the fibers.
Hollow fiber cell culture represents a more in vivo like culture environment, patterned after the vertebrate circulatory system. It is based on the use of hollow fibers, tubular filters shaped like drinking straws of very small diameter, around 200 μm (see Figure 1). They are potted into a cartridge shell so that medium flows through the inside of the fibers while the cells attach and grow on the outer surface of the fibers. Thousands of fibers can be placed inside the cartridge, resulting in a tremendous surface area-to-volume ratio (see Figure 2). The unique properties of hollow fiber cell culture can be summarized as follows:
- High surface area-to-volume ratio (100–200 cm2/mL) permits the culture of cells at in vivo-like cell densities, 108 cells per mL or higher.
- Cells are bound to a 3-D porous support, not a 2-D nonporous impermeable dish. Splitting of the cells is not required. They are free to grow postconfluent.
- The molecular weight cutoff (MWCO) of the fiber can be controlled in manufacturing. Proteins, antibodies, cytokines, and other cell-secreted products can be concentrated in the small volume of the extracapillary space (ECS) and harvested either in a batch mode or continuously.
- Apoptosis is often significantly inhibited, reducing contamination with intracellular proteins and proteases.
- The extremely high cell density supports ease of serum-free and/or simplified medium adaptation. No surfactants, shear-protectants, or antifoams are ever required.
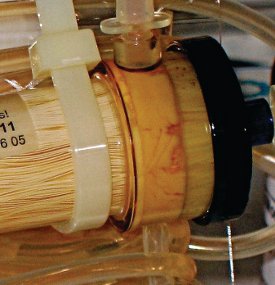
Figure 2 - Hybridoma cells growing at high density in a hollow fiber bioreactor.
In hollow fiber culture, cells do not require splitting, allowing interactions between cells to take place over a period of months if desired. Uniform nutrition and oxygenation permit large numbers of cells to be cultured simultaneously. High cell density facilitates cell-to-cell contact. The MWCO of the fiber also concentrates cytokines involved in the cell-to-cell interaction process. Many of these properties are exploited in specific cellular co-cultivation implementations. Two types of co-cultivation are discussed here: asymmetric cell co-cultivation and one-compartment cell co-cultivation.
Asymmetric cell co-cultivation
One type of cellular co-cultivation is the use of hollow fiber bioreactors to culture endothelial cells on the insides of the fibers and culturing a different cell type on the outside (see Figure 3). Endothelial cells comprise the inner lining of all blood vessels, and the endothelium can actually be considered a discrete organ, rather than simply a cell type. Total mass of the endothelium in an adult human is about 1 kg, providing from 800 to 1000 m2 of surface area. When cultured on the insides of a hollow fiber (the intracapillary space) and exposed to shear stress from medium flow, endothelial cells behave much differently (more in vivo-like) than they do in static, flask culture. Under shear, endothelial cells lay down flat as a monolayer, stop dividing, form tight junctions, and express different genes than when cultured under static conditions in flasks. Differences in shear stress throughout the body may explain different functions of endothelial cells in those areas. Endothelial cells were first cultured on the insides of hollow fibers by Dr. Barbara Ballerman.1 Subsequently, other examples of asymmetric cell co-cultivation have been reported.
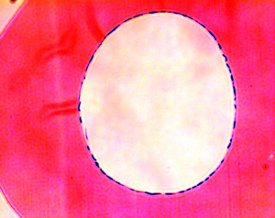
Figure 3 - Cross-section of a single hollow fiber showing human pulmonary endothelial cells growing as a monolayer on the inner surface of the fiber.
Paul Cahill and his group2 cultured bovine aortic endothelial cells on the inside of the fibers and vascular smooth muscle cells on the outside of the fibers (see Figure 4). Since altering the flow rate changed the shear stress, g-protein formation and endothelin receptor expression were directly modulated, even though there was no physical contact between the two cell types. Damir Janigro and his lab3 cultured brain microvascular endothelial cells on the interior of the fibers and astraglial cells on the outside of the fibers. Under these conditions, an active permeability barrier, similar in function to the blood–brain barrier, was created. Projections from the glial cells formed and penetrated the pores of the fiber toward the endothelial cells. In both cases, it was demonstrated that factors are being secreted by the endothelial cells that can affect the function and physiology of other cell types on the outside of the fiber, and also that shear stress applied to the endothelial cells is a critical factor.
Other areas that show promise for this type of asymmetric cell co-cultivation are liver cell culture, where endothelial cells play an important role, and bone marrow co-culture, where endothelial cells may be involved in hematopoietic stem cell differentiation and maturation.
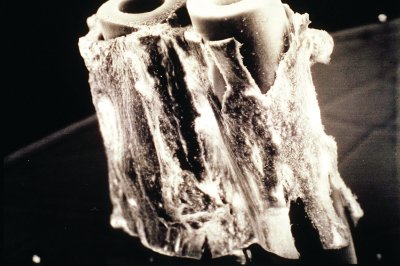
Figure 4 - Scanning electron microscope (SEM) image of vascular smooth muscle growing on the outside of the fiber; bovine aortic endothelial cells are on the inside of the fiber.
One-compartment co-cultivation
Historically, the science of cell biology was intended to reduce complex systems to their most fundamental components in order to better understand those components. However, in this age of Systems Biology, we are better appreciating the implications to research that organisms and tissues are not made of one type of cell and that 3-D structures require complex signaling between multiple types of cells. Hollow fiber cell culture permits the recapitulation of structures containing more than one cell type in a defined, controlled, and more biomimetic, in vivo-like environment. It is one of the few ways to get large numbers of cells in close enough proximity and in high enough densities to observe the interactions between different cell types in an in vitro environment.
This concept of “organ recapitulation” in hollow fiber was first applied by Jorg Gerlach using liver tissue.3 The system used was a complex hollow fiber bioreactor with two different fiber types and three separate bundles of fibers. Liver tissue was simply digested with collagenase, and this mixture of cells was seeded into the hollow fiber bioreactor. Primary function was maintained and supported for four weeks. It appeared that endothelial cells provided a necessary component of the cell mixture and were involved in the formation of 3-D structures.4
Following the same guidelines and principles, FiberCell® Systems (Frederick, MD) performed the same sort of culture, but instead using cells from human placenta.5 The placenta is a tremendous source of stem cells and stem cell support cells, and generates the vast majority of hematopoietic stem cells and other stem cell types for the developing fetus as well as mesenchymal-stromal stem cells. A fresh placenta was perfused with buffer for one hour in order to flush out the cord blood. Then the placenta was perfused with a collagenase solution (Worthington Biochemical Corp., Lakewood, NJ) and the endothelial cells, stromal cells, and a wide variety of other cell types, including stem cells and stem progenitor cells, were collected (see Figure 5).
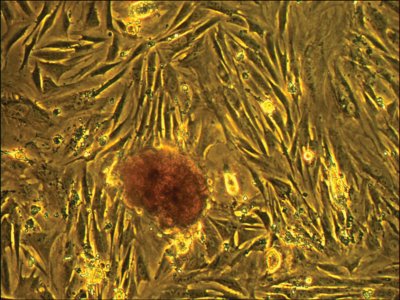
Figure 5 - Placental cells harvested from the hollow fiber bioreactor and placed in a T-75 flask. After three days of flask culture, the adherent cell layer is well established and embryoid bodies are well defined.
The goal of this application note was to evaluate the performance of a crude mixed population of placenta-derived cells to these unique culture conditions. Previous data had shown a significant increase in glucose uptake rate when the 5-kd MWCO cartridge was used as opposed to the 20-kd MWCO cartridge. This is likely due to the retention of cytokines within the extracapillary space facilitating cell-to-cell interactions and consequent metabolic response. During the three-month cultivation period, a significant number of cells and clusters of cells were collected from the cartridge. When these cells were plated in flasks, a certain portion of this population would attach and display interesting morphology, while the embryoid bodies themselves seemed to be more defined and perhaps increase in numbers. The anchorage-dependent cells appeared to be associated in some way with the clustered, embryoid bodies of cells. These clusters of cells stained intensely with a mesenchymal stem cell marker.
These data show that the use of hollow fiber bioreactor cartridges can support the culture of primary human placental-derived cells for extended periods of time, at least three months. During this time, embryoid bodies/cell clusters were harvested and future work aims to further characterize them. This method can act as a replacement for the separation of adherent from nonadherent cell types commonly performed in flask culture, with an additional advantage that larger numbers of cells can be processed at one time. Cell-to-cell interactions, facilitated by the smaller molecular weight cutoff, appear to be important, retaining more cytokines of smaller molecular weight.
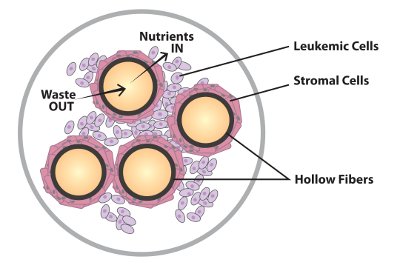
Figure 6 - Stromal cell/suspension cell interaction.
Use of a hollow fiber bioreactor in stromal cell/suspension cell interactions
Perhaps the most rigorous application of hollow fiber systems for cell co-cultivation is in the area of stromal cell/suspension cell interactions (see Figure 6). Contrary to popular belief, it is possible to harvest large numbers of cells from a hollow fiber bioreactor, but the most efficient applications for hollow fiber systems involve longer-term cultures with periodic harvesting over time. There are a couple of recent examples of this with some very interesting results. Dr. Mayasari Lim at University Hospital, Hong Kong, has published an article6 on the co-cultivation of a human bone marrow stromal cell line with a leukemic T cell line. This is a standard protocol generally performed in flask culture, but in this case, Dr. Lim was able to establish the stromal line to fairly high density within one week of culture in the cartridge. Standard mediums were used. Once the glucose uptake rate of the cartridge reached 1 g per day, the leukemic t-cell line was seeded into the cartridge. When co-cultured in flasks, the t-cells underwent a 10× expansion. When cultured in the cartridge, the t-cells underwent a 4000-fold expansion, much more in line with what occurs in vivo.
Hollow fiber bioreactors offer a unique environment for cellular co-cultivation. They represent a 3-D environment similar to the conditions found in vivo, and many parameters, such as oxygenation levels and shear stress, can be controlled. Hollow fiber bioreactors are also an effective means for the generation of conditioned medium for use in stem cell research.
The 3-D environment created within a hollow fiber bioreactor is unique and effective at supporting stroma/suspension co-cultures in sufficient numbers over long periods of time, and has been shown to be potentially clinically useful.
References
- Ballerman, B.; Ott, M. Adhesion and differentiation of endothelial cells by exposure to chronic shear stress: a vascular graft model. Blood Purif.1995, 13, 125–34.
- Redmond, E.; Cahill, P.; et al. Perfused transcapillary smooth muscle and endothelial cell co-culture—a novel in vitro model. In Vitro Cell. Dev. Biol. Sept 1995, 31, 601–9.
- Stanness, K.A.; Westrum, L.E. et al. Morphological and functional characterization of an in vitro blood–brain barrier model. Brain Res. Oct 17, 1997, 771(2), 329–42.
- Zeilinger, K.; Holland, G. et al. Time course of primary liver cell reorganization in three-dimensional high-density bioreactors for extracorporeal liver support: an immunohistochemical and ultrastructural study. Tissue Eng. Jul/Aug 2004, 10(7–8), 1113–24.
- Application note. Placental Co-culture in a Hollow Fiber Bioreactor; www.fibercellsystems.com.
- Binte, S.; Usuludin, M. et al. Co-culture of stromal and erythroleukemia cells in a perfused hollow fiber bioreactor system as an in vitro bone marrow model for myeloid leukemia. Biotech. Bioeng.2012, 109(5).
John J.S. Cadwell is President and CEO, FiberCell® Systems Inc., 905 West 7th St., #334, Frederick, MD 21701, U.S.A.; tel.: 301-471-1269; fax: 301-865-6375; e-mail: [email protected];
www.fibercellsystems.com.