2008 marked the 25th anniversary of inductively coupled plasma-mass spectrometry (ICP-MS). The marriage of ICP and MS resulted in an analytical technique capable of multielemental determinations. This capability opened up larger areas of the periodic table for environmental, forensic, biological, and material science analysis. Since its birth, ICP-MS has evolved rapidly and is at the forefront of elemental and trace metals analysis worldwide.
Background information
Over 25 years ago, mass spectrometry was rarely used for the routine determination of trace elements. There were several issues with the technique that made routine trace analysis impractical at the time. These included unsuitable ion sources, extensive sample preparation, and time-consuming analysis. Prior to ICP-MS, other techniques used to perform elemental analysis included atomic absorption spectroscopy (AAS) and inductively coupled plasma-optical emission spectroscopy (ICP-OES).
AAS uses the absorption of light by atoms to measure their concentrations. Initially, the sample is vaporized in a flame or graphite furnace. The elemental concentration of a sample can be determined as the atoms transition to a higher energy level through the absorption of light. The instrument is calibrated using standard solutions for the elements to be quantitated. However, AAS is subject to certain matrix effects that may require the use of modifiers. Use of the graphite furnace reduces the matrix effects because of the high temperature of the furnace.
ICP-OES is another technique used to determine trace elements in a sample. The ICP excites the atoms and ions in a sample to produce light of wavelengths that are characteristic of each of the elements in the sample. Even though the high temperature of the plasma for all practical purposes eliminates matrix effects, there are interferences caused by the overlapping of the spectral wavelengths of certain elements, which makes it difficult to distinguish these elements in a sample.
Therefore, researchers began the search for a new technique for the determination of trace elements that could address problems experienced by other techniques. Much of the R&D on ICP-MS was performed by researchers Robert S. Houk, Velmer A. Fassel, Gerald D. Fiesch, and Harry J. Svec from Iowa State University (ISU, Ames, IA) and Allen Gray from the University of Surrey (Surrey, U.K.). The objective of their work was to demonstrate the feasibility of ICP-MS for the determination of elemental concentrations and isotopic abundance ratios in solution.1
Several technical problems were encountered by these pioneers during their research, however. The first problem was the hot-gas flow vacuum aperture. They used a small sampling orifice with a diameter of 50 μm, which frequently became clogged with salts. The second problem was electrical arching between the plasma and the sampling orifice. This caused many doubly charged and other spectrally interfering ions in the mass spectrum. The first commercial instrument was introduced in 1983 by MDS Sciex (Concord, Ont., Canada).
The first ICP-MS system
Though much of the early work in ICP-MS was carried out by Houk and Gray, Don J. Douglas, presently a professor at the University of British Columbia (Vancouver, Canada), was the principal scientist at MDS Sciex, and his work made the technique practical. Douglas and his colleagues were able to solve the problems the pioneers were having with the technique at the time. Douglas first used an orifice that was approximately ten times larger than that used by previous researchers to prevent potential clogging. He then developed a cryogenic pumping system that would withdraw the ions in the plasma through the orifice, the interface, and into the mass spectrometer with high fidelity.
Furthermore, additional testing was required to get the system to work, which involved electrical grounding of the plasma source relative to the mass spectrometer and removing radiofrequency interferences from the plasma source. Following this was a second part of the project that involved the analytical problems encountered when measuring liquids other than aqueous standards, which involved handling the chemical and spectral interferences and contamination effects derived from real-world samples.
Just three years after the first paper1 on the use of ICP-MS was published, PerkinElmer (Norwalk, CT)/MDS Sciex introduced the ELAN® 250 (Figure 1), the first commercially available ICP-MS instrument, at the annual Pittsburgh Conference in March 1983. This was the first product of its kind, and it attracted a lot of attention at Pittcon® because of the significant improvement in detection limits and its speed for elemental analysis. Comparable to the size of 2–3 filing cabinets, the ELAN 250 could analyze >90% of the elements in the periodic table in concentrations ranging from 0.5 to 10 parts per billion (ppb).2
A brief overview
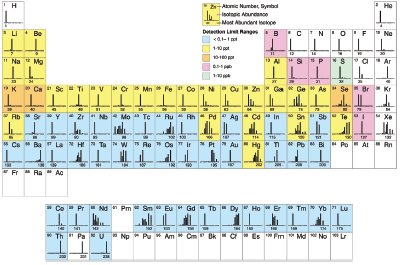
Figure 2 - Elements determined by ICP-MS and approximate detection capability.3 (Reproduced with permission from Ref. 3.)
ICP-MS can analyze liquid and solid samples. An ICP-MS system consists of the following: sample introduction system, ICP torch, interface, vacuum system, lens, quadrupole mass spectrometer, detector, and data management system. First, the sample is introduced into the sample introduction system. The nebulizer, which is the first component of the sample introduction system, converts the sample into small droplets. The small droplets are then passed into the next component of the sample introduction system called the spray chamber. From here, the sample is passed through the ICP torch that contains the plasma, the ion source for ICP-MS. As the sample is ionized in the plasma, it is then passed through an interface into the mass spectrometer. The ions from the sample are then focused through a series of ion lenses and then pass into the quadrupole mass spectrometer, which separates the ions from the sample according to their mass-tocharge ratio. Following the separation, a detector measures the separated ions. Afterward, the data are analyzed by a computerized data system. Figure 2 illustrates the elements determined by ICP-MS and the approximate detection capability.3
The evolution of ICP-MS
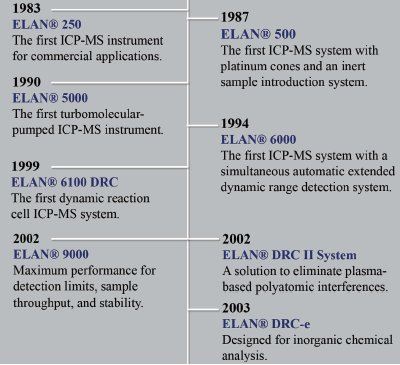
Figure 3 - Evolution of the ELAN family.
Since its introduction in 1983, the ELAN 250 has rapidly evolved over the past 25 years. Figure 3 illustrates the evolution of the ELAN family of instruments. This first ICP-MS instrument required a considerable amount of expertise to operate. Manual adjustments were required of control knobs, lenses, etc. Instrumentation has evolved through continuous efforts up through the 1990s to the point where ICP-MS instruments are now highly automated, stable, and suitable for routine analysis. “The end of 1985 marked the watershed year,” said Dr. Andy Boorn, President of MDS Sciex. At the end of 1985, a booming 30 units were sold. “Wow, this is going to be big,” thought Dr. Boorn. Although more than 900 units are now sold every year, at that time, the level of sales in the first year marked the indication of the true need and future success of ICP-MS.