Ultrahigh-performance liquid chromatography (UHPLC) utilizes sub-2-μm-diam stationary phase particles to provide improved performance over conventional HPLC. This can be realized either in a higher plate number and better resolution, in faster analyses with the same resolution, or some mixture of both benefits.1 UHPLC methods equivalent to conventional HPLC methods but with a 75% savings in analysis time can be easily derived whenever a UHPLC stationary phase is available, with selectivity close to that of the currently used conventional stationary phase.2,3 If the particle size is lowered by half (for example, from 3.5-μm to 1.7-μm particles), then the column length must be lowered by half and the flow rate doubled to provide the same number of theoretical plates and maintain equivalency between the HPLC and UHPLC conditions. The combination of the faster flow over the shorter column is the source of the 75% time savings. (When gradients are used, the times in the gradient table must also be shortened appropriately to maintain equivalency.) The prospect of such large time savings and the associated increase in productivity has created tremendous excitement and has raised performance expectations among HPLC users.
Unfortunately, it is usually not possible to do this simple modification on a conventional HPLC instrument because of three problems: First, with the modification of conditions mentioned previously, the pressure required to achieve the proper flow rate increases by 4×, which is often beyond the pressure limit of a conventional HPLC instrument. Second, the peak width contributions from the column when measured in time are reduced to 25% of what they were originally, but the peak width contribution from the extracolumn parts of the system when measured in time is approx. 70% of what it was originally. (The extracolumn volume is unchanged, but the extracolumn broadening measured in time is reduced at the higher flow rate.) The extracolumn broadening will therefore contribute a much larger fraction to the observed peak widths and will reduce resolution from that of the original separation if no other precautions are taken. Third, since the peaks become one-fourth of their original width, the data collection rate should ideally be four times faster to prevent resolution loss from sampling too slowly. To solve these problems, many users resort to purchasing a new UHPLC instrument; however, a conventional HPLC can be used to produce UHPLC-like separations if these problems can be solved. These issues will be addressed here, beginning with pressure.
Most conventional and UHPLC stationary phase particles are totally porous. Plate generation efficiency is better when smaller-diameter particles are substituted because the solute diffusion distance from the surface into the particle is reduced and mass transfer is improved. However, the pressure required to achieve mobile phase flow varies with the inverse square of the particle diameter; thus using smaller particles rapidly increases the required pressure.
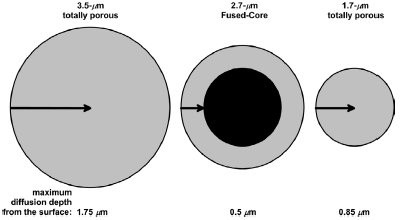
Figure 1 - Comparison of a conventional 3.5-μm totally porous particle, a Fused-Core particle, and a totally porous sub-2-μm particle.
Fused-Core™ (Advanced Materials Technology, Wilmington, DE) particles are designed to achieve high efficiency without requiring sub-2-μm diameters, thereby greatly lowering the pressure required to achieve UHPLC-like performance.4–6 These particles typically have a 2.7-μm overall diameter with a 0.5-μm porous layer over a 1.7-μm solid core. Figure 1 compares particles and shows how the diffusion depth is reduced when access to the particle core is restricted. The sample loading capacity is reduced only 25% by the solid core; therefore 75% of the loading capacity remains. However, the pressure required is 60% less than with sub-2-μm particles. The pressure is low enough that conventional HPLC instruments can be used if the extracolumn broadening and data rate requirements can be managed.
A 4.6 mm i.d. × 100 mm Ascentis Express C18 column (Sigma-Aldrich, Bellefonte, PA) packed with 2.7-μm Fused-Core particles was evaluated on several UHPLC and HPLC instruments. All had UV detectors. The first measurements examined the column characteristics to see if Fused-Core particles can produce plates at rates comparable to totally porous sub-2-μm particles. Also, the column was replaced with a zero-dead-volume union, and the extracolumn contribution to peak width was separately measured at every flow rate.
If the various sources of peak broadening in the system are independent, then the peak width observed in a chromatogram is given by Eq. (1):

where the extracolumn component of peak width has been further subdivided into individual sources of w2 from the injector, connectors, tubes, detector, etc., on the right side of the equation.
Fused-Core column performance in UHPLC instruments
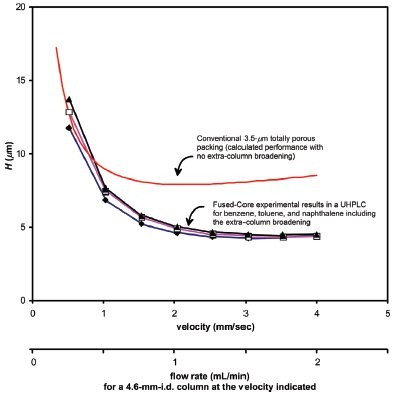
Figure 2 - Experimental plate heights for benzene (♦), toluene (❑), and naphthalene (▲) on a Fused-Core column in a UHPLC system using 50/50 water/acetonitrile at 35 °C, and calculated plate heights for a totally porous 3.5-μm packing (derived from the Knox equation7 with A = 1, B = 2, C = 0.05, and Dm = 2.4 × 10–5 cm2/sec).
Retention and peak widths using a Fused-Core column in two different UHPLC instruments were measured experimentally over a wide range of flow rates. The mobile phase was 50/50 water/acetonitrile; the temperature was 35 °C; and the solutes were benzene (k = 2.6), toluene (k = 4.5), and naphthalene (k = 6.6). In both UHPLC instruments, approx. 23,000 theoretical plates were realized for each solute at optimal velocity. With these retention factors, the van Deemter curves are quite flat at higher-mobile-phase velocities (Figure 2). The optimal plate height (H) was 4.3 μm, or approx. 1.6× the particle diameter. For comparison, the van Deemter performance typical of conventional 3.5-μm particles is also shown in the figure.
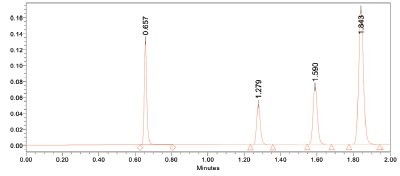
Figure 3 - Separation of uracil, benzene, toluene, and naphthalene (in elution order) using a 4.6 × 100 mm Fused-Core column (Ascentis Express C18) on a UHPLC instrument with 30/70 water/acetonitrile at 35 °C and 1.25 mL/ min. The experimental plate count for the naphthalene peak is 23,781.
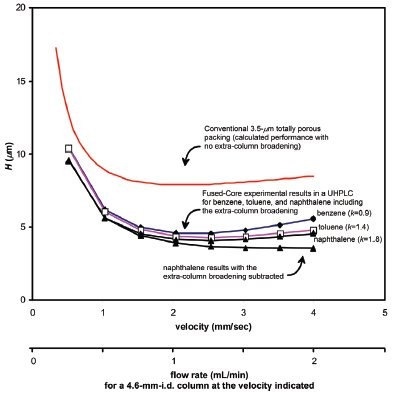
Figure 4 - Experimental plate heights for benzene, toluene, and naphthalene on a Fused-Core column in a UHPLC system using 30/70 water/acetonitrile at 35 °C. With stronger mobile phase and lower retention, extracolumn effects are more apparent than in Figure 2. The lowest curve is for naphthalene with the extracolumn contributions subtracted to indicate the performance of the column separate from the system.
Peak widths naturally get narrower when retention is decreased, but extracolumn broadening remains the same if nothing else is changed. Therefore, the detrimental effects of the system contribute a bigger fraction of the peak width when the retention factor is decreased. For a more stringent test, the mobile phase was made 30/70 water/acetonitrile, also at 35 °C; this reduced the retention factors to 0.9, 1.4, and 1.8, respectively, for the solutes. A typical chromatogram with these conditions is shown in Figure 3, and the corresponding van Deemter curves in Figure 4. The minimum plate height did not change appreciably, and both UHPLC systems provided over 23,000 theoretical plates with this column installed. The optimal flow rate was approx. 1.2 mL/min, and the slopes of the van Deemter curves increased slightly at higher velocities. Even with the demanding conditions of such low solute retention, the improvement over the performance of conventional 3.5-μm totally porous packings is noteworthy. This work verified that the Fused-Core particles produce plates similarly to sub-2-μm totally porous particles. The pressure required to pump mobile phase at 2 mL/min for the 4.6 × 100-mm column with 70% acetonitrile at 35 °C was approx. 220 bar (3200 psi).